Screening of photosensitizers-ATP binding cassette (ABC) transporter interactions in vitro
Abstract
Aim: ATP-binding cassette (ABC) transporters are proteins responsible for the efflux of drug molecules from cancer cells, reducing the efficacy of anti-cancer treatments. This study assesses the susceptibility of a panel of clinically used photosensitizers to be transported by ABC transporters in vitro.
Methods: The involvement of P-glycoprotein (P-gp/ABCB1), breast cancer resistance protein (BCRP/ABCG2), and multidrug resistance-associated protein 1 (MRP1/ABCC1) in the transport of 7 clinically utilized photosensitizers [benzoporphyrin derivative (BPD), temoporfin, redaporfin, talaporfin sodium, rose bengal, methylene blue, and indocyanine green] were investigated using human breast cancer cell lines following well-established protocols. Briefly, parental MCF-7 cells and sublines that overexpress P-gp (MCF-7 TX400), ABCG2 (MCF-7 MX100), or MRP1 (MCF-7/VP) were treated with photosensitizers with and without ABC transporter inhibitors. Intracellular levels of photosensitizers were measured using extraction method and flow cytometry to determine whether the ABC transporters are associated with efflux or uptake of photosensitizers.
Results: The ABCG2 inhibitor (fumitremorgin C) and P-gp inhibitor (valspodar) effectively blocked the transport mediated by ABCG2 and P-gp of rose bengal and BPD. Redaporfin showed increased accumulation in the presence of valspodar with flow cytometry. Interestingly, MCF-7/VP cells were found to have reduced intracellular accumulation of rose bengal, which was restored with MRP1 inhibitor (MK571). The cell viability assay showed photodynamic therapy (PDT) resistance with Redaporfin in P-gp-overexpressing cells, BPD in ABCG2- and P-gp-overexpressing cells, and with Rose bengal in ABCG2-, P-gp- and MRP1-overexpressing cells, respectively. However, no change in intracellular retention was observed for other photosensitizers.
Conclusion: In summary, our study provided new knowledge that temoporfin, talaporfin sodium, methylene blue, and indocyanine green are not substrates of ABCG2, P-gp, or MRP1. Redaporfin is a substrate for P-gp. BPD is a known substrate of ABCG2 and P-gp. Rose bengal is a substrate of ABCG2, P-gp, and MRP1. The results presented here indicate ABC transporter substrate status as a possible cause for cellular resistance to photodynamic therapy with rose bengal, redaporfin, and BPD.
Keywords
INTRODUCTION
Photodynamic therapy (PDT) is a clinically approved treatment modality used to manage neoplastic and nonmalignant diseases[1]. PDT utilizes light of specific wavelengths within the 500-800 nm range to stimulate a light-activable compound known as a photosensitizer (PS)[2]. The activation of photosensitizer leads to the generation of reactive molecular species such as 1O2, H2O2, O2•-, •OH, which induces cytotoxicity in adjacent targets[3,4]. The cytotoxic effects of PDT are governed by various factors such as intracellular accumulation and distribution of the photosensitizer within cells, the spatial arrangement of light, and the diffusion range of active reactive molecular species (< 0.02 μm)[2,3,5,6].
Several emerging photosensitizers are being investigated for the treatment of tumors[6,7]. Many photosensitizers being investigated are chlorins, porphyrins, and dyes. Verteporfin [benzoporphyrin derivative (BPD)], also known as Visudyne, received FDA approval in 2000 for treating wet age-related macular degeneration (AMD)[8]. Recent years have seen several clinical trials exploring its efficacy for treating breast cancer[9,10], locally advanced pancreatic cancer[11], melanoma[12], lung cancer[13], and brain tumors[14]. Foscan® also known as temoporfin (mTHPC), is approved by the European Medicines Agency (EMA)[15] for treating advanced head and neck cancer and is under clinical trials (Phase I/II) for bile duct carcinoma[16,17] and lung cancer[18] in the US. Laserphyrin (talaporfin sodium) is a mono-L-aspartyl chlorin that was approved in Japan in 2004 for PDT of early-stage lung cancer[19] and is undergoing clinical trials for the treatment of unresectable hepatocellular carcinoma[20,21] and liver metastases of colorectal cancer[22]. Redaporfin (LUZ11) received an orphan drug designation from EMA for biliary tract cancer in 2016[23] and is currently undergoing phase I/II trials for advanced head and neck cancer[24]. In addition, due to its fluorescence and phototoxic properties, dyes like methylene blue are under investigation as potential photosensitizers for antibacterial and antitumor treatments[25-27]. Indocyanine green (ICG) at higher concentrations and light doses has also been used for PDT in several preclinical studies and is currently being assessed for use in treating periodontal disease and in diabetic patients with peri-implantitis[28,29].
The growing interest in the efficacy of photosensitizers for anti-cancer treatments raises concerns regarding the potential drug resistance in cancer cells, thus limiting their therapeutic success. One of the primary mechanisms underlying multidrug resistance involves the overexpression of ATP-binding cassette (ABC) transporters in cancer cells, which has been linked with the chemoresistance phenotype in patients[30]. ABC transporters are a family of transporter proteins that are responsible for the efflux of various xenobiotics from the cell to the extracellular space against the concentration gradient through ATP hydrolysis[31,32]. Among 48 identified human transporters, breast cancer resistance protein (BCRP) (encoded by ABCG2),
In this study, we sought to investigate how P-gp, ABCG2, and MRP1 transporters influence the intracellular accumulation of a panel of clinically relevant photosensitizers using MCF-7 sublines overexpressing respective ABC transporters. Cellular uptake of BPD, temoporfin, talaporfin sodium, redaporfin, ICG, methylene blue, and rose bengal was evaluated using a quantitative intracellular photosensitizer accumulation using extraction method, and flow cytometry method using MCF-7 MX100, MCF-7 TX400, and MCF-7 VP cell lines overexpressing ACBG2, P-gp, and MRP1, respectively. The results obtained from the extraction method were confirmed using qualitative flow cytometry. Our findings confirm that BPD transport is observed only in cells that overexpress ABCG2 and P-gp. Notably, the intracellular accumulation of temoporfin remains unaffected by the tested ABC transporters.
Furthermore, our results suggest that the intracellular levels of other tested photosensitizers were unaffected by ABCG2, P-gp, and MRP1, except for rose bengal. Redaporfin might also potentially interact with the
METHODS
Chemicals
BPD was obtained from Adooq Bioscience (CAUSA). Redaporfin and talaporfin sodium were obtained from MedChemExpres (NJ, USA). ICG and temoporfin were obtained from Adooq (CA, USA). Methylene blue and rose bengal were obtained from Sigma-Aldrich (St. Louis, MO, USA). BPD, temoporfin, redaporfin, and ICG were dissolved in DMSO. Talaporfin sodium, rose bengal, and methylene blue were dissolved in phosphate-buffered saline (PBS). The MRP1 inhibitor MK571 was obtained from Sigma
Cell culture
The human breast adenocarcinoma cell line MCF-7 and its sublines overexpressing ABC transporters, including the MCF-7 MX100 subline overexpressing ABCG2 (cultured in 100 nM mitoxantrone), the
Western blotting
Western blot analysis was performed to assess ABC Transporter overexpression. MCF-7 sublines (3.0 × 105 cells) were seeded in 35-mm cell culture dishes and allowed to grow for 24 h. Cellular proteins were extracted for western blotting following established protocols[36]. 20 µg of proteins from lysates were separated on NuPAGETM 4%-12% Bis-Tris gels and transferred onto PVDF membranes (Thermo Fisher). Membranes were blocked with 5% milk-containing Tris-buffered saline and polysorbate 20 (TBST) solution for 1 hour, then probed with antibodies for ABCG2 (Kamiya BioMedical MC-177), P-gp (Thermo Fisher MA1-26528), MRP1 (Kamiya BioMedical MC-162), and β-actin antibodies (Cell Signaling 3700). Chemiluminescence generated using SuperSignal West Pico PLUS (Thermo Fisher, MA, USA) was imaged using Azure 500 imager (Azure Biosystems).
Intracellular photosensitizer accumulation using extraction method
Photosensitizer intracellular accumulation was quantified by adapting the extraction method described previously[36,42]. MCF-7 MX100, TX400, and VP cells were plated in 35-mm petri dishes at a cell density of 3.0 × 105 cells per dish to allow overnight culture at 5% CO2 and 37 °C. The next day, cells were incubated with media (NT), photosensitizer alone (PS), and photosensitizer with respective ABC transporter inhibitor (10 µM FTC for ABCG2; 3 µg/mL Valspodar for P-gp; 25 µM MK571 for MRP1) (PS+I) at fixed photosensitizer concentration (BPD: 2 μM; ICG: 100 μM; methylene blue: 20 μM; rose bengal: 100 μM; temoporfin: 2 μM; redaporfin: 20 μM; and talaporfin sodium: 200 μM) for 1 h. The stock concentration of the photosensitizer was measured by recording the absorbance spectrum of the agent using the spectrophotometer and extrapolating the concentration with molar extinction coefficients [Supplementary Table 2]. After 1 h, the cells were washed twice with PBS (Corning, USA) and incubated with media for 1 h for efflux. The PS+I group was incubated with an inhibitor during the second incubation to ensure transporter inhibition. To quantify the intracellular photosensitizer uptake, cells were washed with PBS and then lysed in RIPA buffer for 30 min (Thermo Fisher Scientific, USA). A spectrophotometer (Synergy Neo2; Biotek, VT, USA) was utilized to acquire fluorescence signal of photosensitizers [BPD: 435/700 nm (Ex/Em); ICG: 710/810 nm; methylene blue: 610/688 nm; rose bengal: 520/575 nm; temoporfin: 422/660 nm; redaporfin: 510/750 nm; and TS: 398/650 nm]. Bicinchoninic acid (BCA) Protein Assay (ThermoFisher Scientific) was used to determine protein concentration in mg/mL. Intracellular photosensitizer concentrations were quantified using appropriate standard curves [Supplementary Figure 1] and then normalized to total protein concentration as determined by the BCA assay. The experimental protocol for the extraction method is summarized in Figure 1. All experiments were performed at least three times in duplicate (N ≥ 3).
Figure 1. Schematic of the intracellular photosensitizer accumulation quantification (Extraction) method. 3.0 × 105 MCF-7 MX 100, MCF-7 TX400, and MCF-7/VP cells were plated in 35-mm dishes in duplicate and incubated overnight. After 24 h, the cells were incubated with the desired photosensitizer with or without ABC transporter inhibitors (ABCG2: FTC, P-gp: valspodar or MRP1: MK571) for 1 h at 37 °C in 5% CO2. Cells were washed with PBS and then incubated for 1 h at 37 °C in a photosensitizer-free medium with or without ABC transporter inhibitors to allow efflux. The cells were subsequently washed and collected for cell lysate preparation. The cell lysates were analyzed using BCA assay for protein quantification. The fluorescence intensity of the experimental groups was recorded using the spectrophotometer at specific Ex/Em wavelengths. Created with BioRender.com. FTC: Fumitremorgin C; PBS: phosphate-buffered saline; ABC: ATP binding cassette; BCA: bicinchoninic acid.
Flow cytometry
Flow cytometry studies followed previously established protocols[36,41]. In brief, MCF-7 cells and sublines
Cell viability assays
Briefly, 1.0 × 104 cells were plated in 96-well black-walled clear well plates (Southernlabware, GA) and allowed to grow overnight. The next day, test photosensitizer was added to the cells at varying concentrations (200 μL/well) and allowed to incubate in the dark for 24 h at 37 °C. Each test concentration was treated in triplicate. On Day 2, the wells were washed with 1× PBS and replaced with fresh media. Following media exchange, the cells were exposed to a set light dose of 5 J/cm2 at excitation wavelengths for respective photosensitizers (BPD: 50 mW/cm2, 690 nm; RB: 20 mW/cm2, 520 nm; Redaporfin: 20 mW/cm2, 520 nm; MB: 50 mW/cm2, 665 nm). Cell viability was measured 24 h post-light activation using Cell-Titer Glo® 2.0 luminescent viability assay (Promega Corporation, USA) using the vendor’s protocol. Cell viability was recorded at twelve different testing concentrations per photosensitizer. All experimental conditions were replicated at least three times in triplicates (N ≥ 3).
Statistical analyses
All experiments were performed at least in triplicates. Results are shown with mean ± the standard error of the mean (SEM). Statistical analyses were performed using GraphPad Prism software. One-way analysis of variance (ANOVA) statistical tests and appropriate post hoc analyses were applied to avoid type I errors.
RESULTS
ABC transporter overexpression in MCF-7 breast cancer sublines
To assess the impact of ABC transporter on the accumulation of photosensitizers, uptake studies using extraction and flow cytometry methods were performed with MCF-7 breast cancer sublines overexpressing ABCG2, P-gp, and MRP1. Western blot analysis confirmed the overexpression of ABCG2, P-gp, and MRP1 in MCF-7 MX100, MCF-7 TX400, and MCF-7/VP cell lines, respectively. MCF-7 parental cells exhibit no expression of the transporter proteins [Figure 2].
Figure 2. Western blot analysis of ABGC2, P-gp, and MRP1 expression in MCF-7 (parent cell line), MCF-7 MX100, MCF-7 TX400, and MCF-7/VP cells. Whole-cell extracts were collected and analyzed using Western blot. 20 µg of the whole cell extract was loaded in each lane. β-Actin was used as a loading control. ABC transporter selective cell lines show increased expression of respective transporters compared to the parental (control) cell line. ABC: ATP binding cassette.
Quantifying ABC transporter-mediated intracellular accumulation of photosensitizers in MCF-7 sublines
To evaluate the effect of the ABC transporter on the photosensitizer concentration in the cells, uptake studies were conducted using a panel of MCF-7 sublines that overexpressed ABCG2, P-gp, and MRP1. The intracellular fluorescence of photosensitizers was measured at specific excitation and emission wavelengths [Figure 3]. Intracellular photosensitizer accumulation for experimental groups (NT, PS, and PS+I) was quantified using fluorescence intensity standard curves [Supplementary Figure 1].
Figure 3. Normalized absorbance (blue) and fluorescence (red) spectra of (A) BPD, (B) temoporfin, (C) redaporfin, (D) talaporfin sodium, (E) rose bengal, (F) methylene blue and (G) ICG used in the study. The absorbance spectrum of the test photosensitizers was recorded using a spectrophotometer. The photosensitizers were excited at respective Soret and Q bands to record the emission spectrum of the photosensitizers. BPD Ex: 435 nm; Temoporfin Ex: 422 nm; Redaporfin Ex: 510 nm; Talaporfin sodium Ex: 398 nm; Rose bengal Ex: 520 nm; Methylene blue Ex: 610 nm; ICG Ex: 730 nm. Respective excitation wavelengths at the Soret or Q band were chosen to avoid overlap between the excitation and the emission wavelengths while recording the fluorescence intensity during extraction experiments. BPD: Benzoporphyrin derivative; ICG: indocyanine green.
In ABCG2-overexpressing cells [Figure 4], ABCG2 inhibitor FTC notably augmented the intracellular accumulation of BPD [Figure 4A] and rose bengal [Figure 4E]. However, no significant difference was observed in the intracellular fluorescence of temoporfin, talaporfin sodium, redaporfin, and ICG [Figure 4B-D and F-G]. The intracellular accumulation of methylene blue decreased significantly in the presence of FTC, suggesting competitive inhibition between methylene blue and FTC. Nevertheless, incubation with Ko143, a known ABCG2 inhibitor, showed no change in intracellular methylene blue levels between the PS and PS+I groups, suggesting no interaction between methylene blue and ABCG2 [Supplementary Figure 2]. The results suggest that BPD and rose bengal are substrates of the ABCG2 transporter.
Figure 4. Intracellular levels of photosensitizers in MCF-7 MX100 (ABCG2-overexpressing) cell line using extraction method. Intracellular levels of the photosensitizers from cell lysates were determined by the fluorescence signals of the photosensitizer. BPD and rose bengal show a significant increase in accumulation in MCF-7 MX100 cells in the presence of ABCG2 inhibitor (FTC). The fmole/mg protein of photosensitizer is plotted for all the experimental groups. (A) Benzoporphyrin derivative; (B) Temoporfin; (C) Redaporfin; (D) Talaporfin sodium; (E) Rose Bengal; (F) Methylene blue; (G) Indocyanine green. (*P < 0.05, **P < 0.01, ***P < 0.001; One-way ANOVA Tukey’s range test) (N = 3-6). NT: not treated control; PS: photosensitizer only; PS+FTC: photosensitizer + inhibitor; ns: non-significant; BPD: benzoporphyrin derivative; FTC: fumitremorgin C.
In P-gp overexpressing MCF-7 TX400 cells, adding the P-gp inhibitor significantly increased the intracellular accumulation of BPD compared to the respective PS group [Figure 5A]. No significant increase in photosensitizer accumulation was observed for temoporfin, redaporfin, talaporfin sodium, methylene blue, and ICG [Figure 5B-F]. The intracellular accumulation of rose bengal showed a significant difference compared to the PS group [Figure 5G]. The results obtained with extraction studies with MCF-7 TX400 cells suggest the potential interaction of BPD and rose bengal with the P-gp transporter.
Figure 5. Intracellular levels of photosensitizers in MCF-7 TX400 (P-gp overexpressing) cell line using extraction method. Intracellular levels of the photosensitizers from cell lysates were determined by the fluorescence signals of the photosensitizer. BPD and rose bengal show a significant increase in accumulation in MCF-7 TX400 cells in the presence of a P-gp inhibitor (valspodar). The fmole/mg protein of the photosensitizer was plotted for all the experimental groups. (A) Benzoporhyrin derivative; (B) Temoporfin; (C) Redaporfin; (D) Talaporfin sodium; (E) Methylene blue; (F) Indocyanine green; (G) Rose bengal. *P < 0.05, **P < 0.01, ***P < 0.001; One-way ANOVA Tukey’s range test (N = 3-5). NT: Not treated, control; PS: photosensitizer only; PS+Valspodar: photosensitizer + inhibitor; ns: non-significant; BPD: benzoporphyrin derivative.
For the MRP1-overexpressing cell line, the addition of MK571 (MRP1 inhibitor) led to no significant photosensitizer accumulation in the presence and absence of MK571 for BPD and temoporfin [Figure 6A and B]. The results obtained for redaporfin, talaporfin sodium, methylene blue, and ICG showed no significant photosensitizer accumulation in the presence of the MK571 inhibitor compared to the group without the inhibitor [Figure 6C-F]. A significant accumulation of rose bengal was observed in the PS+I group compared to the PS group [Figure 6G]. These results suggest that only BPD and rose bengal are substrates of ACBG2 and P-gp.
Figure 6. Intracellular levels of photosensitizers in MRP1-overexpressing MCF-7/VP cell line using extraction method. Intracellular levels of the photosensitizers from cell lysates were determined by the fluorescence signals of the photosensitizer. Rose bengal shows a significant increase in accumulation in MCF-7/VP cells in the presence of MRP1 inhibitor (MK571). The fmole/mg protein of photosensitizer is plotted for all the experimental groups. (A) Benzoporhyrin derivative; (B) Temoporfin; (C) Redaporfin; (D) Talaporfin sodium; (E) Methylene blue; (F) Indocyanine green; (G) Rose bengal. *P < 0.05, **P < 0.01, ***P < 0.001; One-way ANOVA Tukey’s range test
ABC transporter-mediated effects on photosensitizer accumulation in MCF-7 breast cancer sublines investigated by flow cytometry
Flow cytometry was performed to confirm the results obtained with the extraction method approach and assess the effect of ABC transporters on photosensitizer accumulation. The fluorescence signal of photosensitizers was recorded using flow cytometry. In MCF-7 MX100 cells, the addition of FTC resulted in increased fluorescence of both BPD and rose bengal [Figure 7]. MCF-7 MX100 cells were also found to accumulate less methylene blue than the PS group when incubated with the FTC inhibitor. Notably, FTC led to a slight decrease in intracellular methylene blue fluorescence in the parental cell line, indicating limited transportation of methylene blue by ABCG2. A similar trend was also observed with the extraction studies performed with methylene blue and FTC. Intracellular levels of temoporfin, redaporfin, talaporfin sodium, and ICG remained largely unaffected by the presence of FTC in both MCF-7 MX100 and parental cells [Figure 7].
Figure 7. ABCG2-overexpressing cells transport selected photosensitizers (BPD and Rose Bengal). Flow cytometry was performed to assess the intracellular fluorescence of photosensitizer. The raw mean fluorescence intensity values are reported for individual histograms. BPD and Rose bengal show higher uptake in the presence of the ABCG2 inhibitor, FTC. NT: Not treated, control; PS: photosensitizer only; PS+Inhibitor: photosensitizer + FTC; BPD: benzoporphyrin derivative; FTC: fumitremorgin C.
In Figure 8, upon treatment of P-gp-overexpressing with 2 μM of BPD, a noticeable decrease in fluorescence intensity was observed in the PS group. However, when cells were treated with BPD and valspodar, a notable increase in BPD fluorescence was observed in TX400 cells. An increase in rose bengal intracellular fluorescence was also observed in the PS+I group compared to the PS group. However, this trend was not evident in the parental cell line. Thus, elevated levels of valspodar-inhibitable efflux of both BPD and rose bengal were observed. Valspodar also increased the intracellular fluorescence intensity of redaporfin in
Figure 8. P-gp-overexpressing cells transport selected photosensitizers (BPD, Rose Bengal, and Redaporfin*). Flow cytometry was performed to assess the intracellular fluorescence of photosensitizer. The raw mean fluorescence intensity values are reported for individual histograms. BPD, redaporfin, and rose bengal show higher uptake in the presence of P-gp inhibitor valspodar. Heterogeneity in the single-cell population for the redaporfin-only group was observed in MCF-7 TX400 cells. NT: Not treated, control; PS: photosensitizer only; PS+Inhibitor: photosensitizer + valspodar; BPD: benzoporphyrin derivative.
MCF-7/VP cells demonstrated appreciable MRP1-mediated transport of rose bengal, as demonstrated by the PS (pink line) and PS+I group (green line) [Figure 9]. However, only a slight increase in the P+I group was observed in the MCF-7 parental cells. MRP1 transported none of the tested photosensitizers besides rose bengal (Figure 9, column 2).
Figure 9. MRP1 transports a selected photosensitizer (Rose Bengal). Flow cytometry was performed to assess the intracellular fluorescence of photosensitizer. The raw mean fluorescence intensity values are reported for individual histograms. Rose bengal shows higher uptake in the presence of MRP1 inhibitor MK571. NT: Not treated, control; PS: photosensitizer only; PS+Inhibitor: photosensitizer + MK571.
These results indicate that ABCG2 and P-gp translocate BPD but not MRP1 as expected. Rose bengal, on the other hand, is a substrate for ABCG2, P-gp, and MRP1 transporter. However, none of the other tested photosensitizers showed ABCG2, P-gp, and MRP1-mediated transport in MCF-7 cell lines overexpressing ABC transporters. The normalized flow cytometry fluorescence intensities are reported in Supplementary Table 3.
ABC transporter-photosensitizer interactions affect PDT-mediated phototoxicity
To assess whether ABC transporter-mediated translocation of photosensitizers observed with extraction and flowcytometry studies for BPD, rose bengal, and redaporfin would result in resistance from PDT, cell viability assays were performed using MCF-7 sublines and parental cells [Figure 10]. The IC50 values obtained for respective cell lines are summarized in Figure 10E. In agreement with the results obtained by flow cytometry, redaporfin showed resistance to PDT in MCF-7 TX400 cells overexpressing P-gp. The IC50 of redaporfin in MCF-7 TX400 cells (182 20.2 M) was determined to be significantly higher than the IC50 of redaporfin in parental cells (0.559 0.069 M). No significant difference in IC50 was observed in ABCG2 and MRP1 cells compared to parental cells for redaporfin.
Figure 10. Photosensitizers that are ABC transporter substrates are resistant to PDT. Cell viability assays were performed 24 h post-PDT for (A) BPD, (B) Methylene Blue, (C) Redaporfin, and (D) Rose Bengal; (E) The IC50 (reported in μM) for each photosensitizer for the respective cell line is summarized in the table. ABC: ATP binding cassette; PDT: photodynamic therapy; BPD: benzoporphyrin derivative.
In contrast, methylene blue did not show significant PDT resistance in any of the MCF-7 sublines overexpressing ABCG2, P-gp, or MRP1. The IC50 values for methylene blue were similar between the parental cells and the ABC transporter-overexpressing sublines. However, PDT resistance was observed in all MCF-7 sublines when treated with rose bengal. A significant increase in IC50 concentrations was observed for rose bengal in MCF-7 MX100, MCF-7 TX400, and MCF-7/VP cells compared to the parental cells.
DISCUSSION
Drug resistance continues to be a leading cause of treatment failure and remains a significant challenge in cancer therapies. Multidrug resistance (MDR) involves various intrinsic and acquired factors that help evade the cytotoxic effects of structurally and functionally unrelated drugs[43]. Previous studies have demonstrated a positive correlation between ABC transporter co-expression and decreased relapse-free survival in cancer patients[44-46]. PDT is a promising approach to address drug resistance in cancer[47]. Various studies have examined the effectiveness of PDT in overcoming MDR and resensitizing tumor cells to treatment. For instance, previous studies have shown the efficacy of temoporfin-mediated PDT against
Given the limited data on the impact of ABC drug transporters on photosensitizer efflux, the ability of ABCG2, P-gp, and MRP1 to decrease the intracellular accumulation was determined for a panel of clinically relevant photosensitizers: BPD, temoporfin, redaporfin, talaporfin sodium, rose bengal, methylene blue, and ICG using both quantitative (extraction) and qualitative (flow cytometry) methods. The results obtained with the optimized extraction method for BPD (known substrate for ACBG2 and P-gp, but not MRP1) and temoporfin (not a substrate of ABCG2, P-gp, and MRP1) were consistent with previous findings[36,41]. No significant increase in fluorescence intensity of temoporfin was observed in the panel of ABC transporter-overexpressing cells in the presence of respective inhibitors measured using flow cytometry. BPD, as expected, exhibited increased intracellular fluorescence intensity in ABCG2- and P-gp-overexpressing cells in the presence of FTC and valspodar inhibitors, respectively, demonstrating efflux of BPD through these transporters.
In the presence of FTC inhibitor, cells overexpressing ABCG2 also showed increased fluorescence intensity of rose bengal compared to parental cells. While our observations are in agreement with previous findings[36,41], this study provides new knowledge that redaporfin, talaporfin sodium, and ICG are not substrates of ABCG2. However, the intracellular levels of methylene blue decreased in ABCG2-overexpressing cells in the presence of FTC inhibitor. A similar trend was also observed while measuring the fluorescence intensity of methylene blue in MCF-7 MX100 cells in the presence of FTC with flow cytometry. FTC is the first and widely known ABCG2 inhibitor to modulate drug resistance in cancer therapies. However, FTC is precluded from clinical use due to off-target effects that lead to undesirable neurotoxicity[51,52]. The significant decrease in methylene blue accumulation in the presence of FTC compared to the methylene blue-only group suggests potential off-target interactions with other cellular components that might affect MB uptake, thereby reducing methylene blue uptake in the presence of FTC. On the other hand, extraction experiments with a more potent and specific ABCG2 inhibitor, Ko143, showed no significant difference in methylene blue accumulation between methylene blue-only and methylene blue+Ko143 groups [Supplementary Figure 2].
The interaction of the tested panel of photosensitizers with P-gp and MRP1 transporters was also explored. None of the photosensitizers except rose bengal were found to be substrates of P-gp. Our results with flow cytometry data for redaporfin show a five-fold increase in fluorescence intensity with valspodar in P-gp-overexpressing cells. However, the extraction results did not show this trend [Figure 5C]. Additionally, the broader histogram of fluorescence intensity distribution for the PS groups for MCF-7 TX400 cells indicates a heterogeneous drug uptake profile within the cell population.
In addition to PDT, photosensitizers are also being explored as photodiagnostic agents for fluorescence-guided interventions like fluorescence-guided surgery (FGS) applications in the clinic[53]. Table 1 summarizes the ABC transporter substrate status of clinically relevant photosensitizers. Our results indicate that in tumors expressing P-gp, ABCG2, and MRP1, fluorescence imaging or PDT of tumors could potentially be less effective with photosensitizers that are substrates of ABC transporters.
ABC transporter substrate status of clinically relevant photosensitizers
Photosensitizers | Substrate of ACBG2 | Substrate of P-gp | Substrate of MRP1 | Ref. |
Rose bengal | Yes | Yes | Yes | |
BPD | Yes | Yes | No | [36,54] |
Temoporfin/Foscan® | No | No | No | [41,55] |
Methylene blue | No | No | No | |
Redaporfin | No | Yes | No | |
Talaporfin sodium | No | No | No | |
Indocyanine green | No | No | No | |
5-ALA/PpIX | Yes | No | No | [41,56] |
Chlorin-e6 | Yes | No | No | [40,41] |
Pheorphorbide a | Yes | No | No | [38,39,41] |
MPPa | Yes | No | No | [41] |
HpD | No | No | No | [41] |
Although the results from the in vitro assays from the study confirm the impact of ABC transporter inhibition on the accumulation and efflux of the photosensitizers, further investigations employing molecular docking analysis are warranted to understand potential interactions between rose bengal, redaporfin, and the substrate binding sites of ABCG2, P-gp, and MRP1. Given the incomplete understanding of transporter substrate interaction, employing computational models to predict interaction probabilities of photosensitizers with ABC efflux transporters would provide insights that can inform the development of effective PDT strategies for MDR in cancer patients[57-59].
Thus, photosensitizers that are substrates of ABC transporters may be less effective in treating cancers expressing these transporters. We have also observed that resistance to redaporfin-mediated photodynamic therapy (PDT) increased in cells expressing P-gp, as indicated by a significantly higher IC50 compared to parental cells [Figure 10]. This observation was consistent with flow cytometry results and suggests that
Alternatively, our previous findings have shown that light activation of photosensitizers-substrates could affect ABC transporter protein expression, ATPase activity, and intracellular mitochondrial ATP levels in vitro and improve irinotecan drug accumulation in vitro and in tumor tissues[42,59,67]. Further studies are needed to confirm the PDT efficacy of photosensitizers that are not substrates of ABC transporters, which can escape efflux by these transporters and circumvent drug resistance.
It is important to note that MDR is a complex phenomenon caused by multiple mechanisms that build a complex network of molecular mechanisms mediating the MDR phenotype. Despite temoporfin and talaporfin sodium not being a substrate of ABC transporters, resistance to PDT with the agents in cancer cells has been observed, possibly due to mechanisms such as loss of p53 function and Ras expression[68-71]. Thus, a comprehensive understanding of drug resistance mechanisms is needed to design effective treatment strategies for cancer.
In summary, we have shown that photosensitizers BPD and rose bengal are transported by ABCG2 and
DECLARATIONS
Authors’ contributions
Wrote the manuscript: all the authors
Designed experiments and conceptualization, prepared the manuscript, and performed visualization: Vig S, Huang HC
Performed experiments, analyzed data, and made the figures: Vig S
Performed investigation: Srivastava P, Rahman I
Managed project administration and supervision: Huang HC, Uusimaa P
Performed methodology: Jaranson R, Dasgupta A
Provided administrative, technical, and material support and contributed to the figures: Perttilä R
All the authors contributed to editing the final manuscript and approved the final version of the manuscript.
Availability of data and materials
Data available within the article and the Supplementary Materials.
Financial support and sponsorship
Huang HC acknowledges support from the US NIH Trailblazer Award (NIBIB R21EB028508), US National Science Foundation (CBET-2030253, CBET-2037815), the US NIH (R01CA260340, R01CA256710) and Modulight Corporation. I.R. is supported by the NCI-UMD partnership for integrative cancer research fellowship. Rahman I and Dasgupta A are supported by the University of Maryland ASPIRE Fellowship. Vig S is supported by the MPower Fellowship.
Conflicts of interest
Uusimaa P is a co-founder of and has equity in Modulight Corporation, Finland. Huang HC has research agreements with Modulight Corporation, Finland. The remaining authors declared that there are no conflicts of interest.
Ethical approval and consent to participate
Not applicable.
Consent for publication
Not applicable
Copyright
© The Author(s) 2024.
Supplementary Materials
REFERENCES
1. Obaid G, Celli JP, Broekgaarden M, et al. Engineering photodynamics for treatment, priming and imaging. Nat Rev Bioeng 2024;2:752-69.
2. Dolmans DEJGJ, Fukumura D, Jain RK. Photodynamic therapy for cancer. Nat Rev Cancer 2003;3:380-7.
3. Celli JP, Spring BQ, Rizvi I, et al. Imaging and photodynamic therapy: mechanisms, monitoring, and optimization. Chem Rev 2010;110:2795-838.
5. Ethirajan M, Chen Y, Joshi P, Pandey RK. The role of porphyrin chemistry in tumor imaging and photodynamic therapy. Chem Soc Rev 2011;40:340-62.
6. Dougherty TJ, Gomer CJ, Henderson BW, et al. Photodynamic therapy. J Natl Cancer Inst 1998;90:889-905.
7. Abrahamse H, Hamblin MR. New photosensitizers for photodynamic therapy. Biochem J 2016;473:347-64.
9. ClinicalTrials.gov. Treatment of primary breast cancer using PDT. Available from: https://clinicaltrials.gov/study/NCT02872064. [Last accessed on 13 Sep 2024].
10. ClinicalTrials.gov. An open label, phase II trial of continuous low-irradiance photodynamic therapy (CLIPT) using Verteporfin (Visudyne®) for the treatment of cutaneous metastases of breast cancer. Available from: https://clinicaltrials.gov/study/NCT02939274. [Last accessed on 13 Sep 2024].
11. ClinicalTrials.gov. Ultrasound-guided verteporfin photodynamic therapy for the treatment of unresectable solid pancreatic tumors or advanced pancreatic cancer, VERTPAC-02 study. Available from: https://clinicaltrials.gov/study/NCT03033225. [Last accessed on 13 Sep 2024].
12. ClinicalTrials.gov. Photodynamic therapy in treating patients with stage III or stage IV melanoma. Available from: https://clinicaltrials.gov/study/NCT00007969. [Last accessed on 13 Sep 2024].
13. ClinicalTrials.gov. Interstitial photodynamic therapy following palliative radiotherapy in treating patients with inoperable malignant central airway obstruction. Available from: https://clinicaltrials.gov/study/NCT06306638. [Last accessed on 13 Sep 2024].
14. ClinicalTrials.gov. Verteporfin for the treatment of recurrent high-grade EGFR-mutated glioblastoma. Available from: https://clinicaltrials.gov/study/NCT04590664. [Last accessed on 13 Sep 2024].
15. European Medicines Agency. Foscan. Available from: https://www.ema.europa.eu/en/medicines/human/EPAR/foscan. [Last accessed on 13 Sep 2024].
16. ClinicalTrials.gov. Single-arm study of photodynamic laser therapy using foscan for non-curatively-resectable bile duct carcinoma. Available from: https://clinicaltrials.gov/study/NCT01016002. [Last accessed on 13 Sep 2024].
17. ClinicalTrials.gov. Safety and tumoricidal effect of low dose foscan PDT in Patients with inoperable bile duct cancers (PDT). Available from: https://clinicaltrials.gov/study/NCT03003065. [Last accessed on 13 Sep 2024].
18. ClinicalTrials.gov. Photodynamic therapy during surgery in treating patients with non-small cell lung cancer that can be removed by surgery. Available from: https://clinicaltrials.gov/study/NCT01854684. [Last accessed on 13 Sep 2024].
19. Wang S, Bromley E, Xu L, Chen JC, Keltner L. Talaporfin sodium. Expert Opin Pharmacother 2010;11:133-40.
20. ClinicalTrials.gov. A phase 3 study of talaporfin sodium and interstitial light emitting diodes treating hepatocellular carcinoma (HCC). Available from: https://clinicaltrials.gov/study/NCT00355355. [Last accessed on 13 Sep 2024].
21. ClinicalTrials.gov. Tumor ablation with talaporfin sodium and interstitial light emitting diodes treating hepatocellular carcinoma (HCC). Available from: https://clinicaltrials.gov/study/NCT00122876. [Last accessed on 13 Sep 2024].
22. ClinicalTrials.gov. Photodynamic therapy with talaporfin sodium (LS11) in treating patients with refractory colorectal liver metastases. Available from: https://clinicaltrials.gov/study/NCT00068068. [Last accessed on 13 Sep 2024].
23. European Medicines Agency. EU/3/22/2602 - orphan designation for the treatment of biliary tract cancer. 2022. Available from: https://www.ema.europa.eu/en/medicines/human/orphan-designations/eu-3-15-1470. [Last accessed on 13 Sep 2024].
24. Santos LL, Oliveira J, Monteiro E, Santos J, Sarmento C. Treatment of head and neck cancer with photodynamic therapy with redaporfin: a clinical case report. Case Rep Oncol 2018;11:769-76.
25. Dos Santos AF, Terra LF, Wailemann RA, et al. Methylene blue photodynamic therapy induces selective and massive cell death in human breast cancer cells. BMC Cancer 2017;17:194.
26. Tardivo JP, Del Giglio A, de Oliveira CS, et al. Methylene blue in photodynamic therapy: From basic mechanisms to clinical applications. Photodiagnosis Photodyn Ther 2005;2:175-91.
27. Fathy G, Asaad MK, Rasheed HM. Daylight photodynamic therapy with methylene blue in plane warts: a randomized double-blind placebo-controlled study. Photodermatol Photoimmunol Photomed 2017;33:185-92.
28. ClinicalTrials.gov. ICG-PDT, periimplantitis, diabetes mellitus. Available from: https://clinicaltrials.gov/study/NCT04833569. [Last accessed on 13 Sep 2024].
29. ClinicalTrials.gov. Single session of antimicrobial photodynamic therapy using indocyanine green. Available from: https://clinicaltrials.gov/study/NCT02043340. [Last accessed on 13 Sep 2024].
30. Leonard GD, Fojo T, Bates SE. The role of ABC transporters in clinical practice. Oncologist 2003;8:411-24.
31. Kim Y, Chen J. Molecular structure of human P-glycoprotein in the ATP-bound, outward-facing conformation. Science 2018;359:915-9.
32. Manolaridis I, Jackson SM, Taylor NMI, Kowal J, Stahlberg H, Locher KP. Cryo-EM structures of a human ABCG2 mutant trapped in ATP-bound and substrate-bound states. Nature 2018;563:426-30.
33. Schinkel AH, Jonker JW. Mammalian drug efflux transporters of the ATP binding cassette (ABC) family: an overview. Adv Drug Deliv Rev 2003;55:3-29.
34. Gottesman MM, Lavi O, Hall MD, Gillet JP. Toward a better understanding of the complexity of cancer drug resistance. Annu Rev Pharmacol Toxicol 2016;56:85-102.
35. Tamaki A, Ierano C, Szakacs G, Robey RW, Bates SE. The controversial role of ABC transporters in clinical oncology. Essays Biochem 2011;50:209-32.
36. Baglo Y, Liang BJ, Robey RW, Ambudkar SV, Gottesman MM, Huang HC. Porphyrin-lipid assemblies and nanovesicles overcome ABC transporter-mediated photodynamic therapy resistance in cancer cells. Cancer Lett 2019;457:110-8.
37. Li W, Zhang WJ, Ohnishi K, Yamada I, Ohno R, Hashimoto K. 5-Aminolaevulinic acid-mediated photodynamic therapy in multidrug resistant leukemia cells. J Photochem Photobiol B 2001;60:79-86.
38. Robey RW, Steadman K, Polgar O, et al. Pheophorbide a is a specific probe for ABCG2 function and inhibition. Cancer Res 2004;64:1242-6.
39. Jonker JW, Buitelaar M, Wagenaar E, et al. The breast cancer resistance protein protects against a major chlorophyll-derived dietary phototoxin and protoporphyria. Proc Natl Acad Sci U S A 2002;99:15649-54.
40. Merlin JL, Gautier H, Barberi-Heyob M, Teiten MH, Guillemin F. The multidrug resistance modulator SDZ-PSC 833 potentiates the photodynamic activity of chlorin e6 independently of P-glycoprotein in multidrug resistant human breast adenocarcinoma cells. Int J Oncol 2003;22:733-9.
41. Robey RW, Steadman K, Polgar O, Bates SE. ABCG2-mediated transport of photosensitizers: potential impact on photodynamic therapy. Cancer Biol Ther 2005;4:187-94.
42. Huang HC, Mallidi S, Liu J, et al. Photodynamic therapy synergizes with irinotecan to overcome compensatory mechanisms and improve treatment outcomes in pancreatic cancer. Cancer Res 2016;76:1066-77.
43. Aniogo EC, Plackal Adimuriyil George B, Abrahamse H. The role of photodynamic therapy on multidrug resistant breast cancer. Cancer Cell Int 2019;19:91.
44. Bartholomae S, Gruhn B, Debatin KM, et al. Co-expression of multiple ABC-transporters is strongly associated with treatment response in childhood acute myeloid leukemia. Pediatr Blood Cancer 2016;63:242-7.
45. Ween MP, Armstrong MA, Oehler MK, Ricciardelli C. The role of ABC transporters in ovarian cancer progression and chemoresistance. Crit Rev Oncol Hematol 2015;96:220-56.
46. Hlavata I, Mohelnikova-Duchonova B, Vaclavikova R, et al. The role of ABC transporters in progression and clinical outcome of colorectal cancer. Mutagenesis 2012;27:187-96.
47. Casas A, Di Venosa G, Hasan T, Al Batlle. Mechanisms of resistance to photodynamic therapy. Curr Med Chem 2011;18:2486-515.
48. Kukcinaviciute E, Sasnauskiene A, Dabkeviciene D, Kirveliene V, Jonusiene V. Effect of mTHPC-mediated photodynamic therapy on 5-fluorouracil resistant human colorectal cancer cells. Photochem Photobiol Sci 2017;16:1063-70.
49. Kim JH, Park JM, Roh YJ, Kim IW, Hasan T, Choi MG. Enhanced efficacy of photodynamic therapy by inhibiting ABCG2 in colon cancers. BMC Cancer 2015;15:504.
50. Liu FS. Mechanisms of chemotherapeutic drug resistance in cancer therapy--a quick review. Taiwan J Obstet Gynecol 2009;48:239-44.
51. Yuan Y, Chen K, Chen X, et al. Fumitremorgin C attenuates osteoclast formation and function via suppressing RANKL-induced signaling pathways. Front Pharmacol 2020;11:238.
52. Jackson SM, Manolaridis I, Kowal J, et al. Structural basis of small-molecule inhibition of human multidrug transporter ABCG2. Nat Struct Mol Biol 2018;25:333-40.
53. Nagaya T, Nakamura YA, Choyke PL, Kobayashi H. Fluorescence-guided surgery. Front Oncol 2017;7:314.
54. Liu W, Baer MR, Bowman MJ, et al. The tyrosine kinase inhibitor imatinib mesylate enhances the efficacy of photodynamic therapy by inhibiting ABCG2. Clin Cancer Res 2007;13:2463-70.
55. Teiten MH, Bezdetnaya L, Merlin JL, et al. Effect of meta-tetra(hydroxyphenyl)chlorin (mTHPC)-mediated photodynamic therapy on sensitive and multidrug-resistant human breast cancer cells. J Photochem Photobiol B 2001;62:146-52.
56. Tsai T, Hong RL, Tsai JC, Lou PJ, Ling IF, Chen CT. Effect of 5-aminolevulinic acid-mediated photodynamic therapy on MCF-7 and MCF-7/ADR cells. Lasers Surg Med 2004;34:62-72.
57. Sajid A, Rahman H, Ambudkar SV. Advances in the structure, mechanism and targeting of chemoresistance-linked ABC transporters. Nat Rev Cancer 2023;23:762-79.
58. Montanari F, Ecker GF. Prediction of drug-ABC-transporter interaction - recent advances and future challenges. Adv Drug Deliv Rev 2015;86:17-26.
59. Liang BJ, Lusvarghi S, Ambudkar SV, Huang HC. Mechanistic insights into photodynamic regulation of adenosine 5’-triphosphate-binding cassette drug transporters. ACS Pharmacol Transl Sci 2021;4:1578-87.
60. Palasuberniam P, Yang X, Kraus D, Jones P, Myers KA, Chen B. ABCG2 transporter inhibitor restores the sensitivity of triple negative breast cancer cells to aminolevulinic acid-mediated photodynamic therapy. Sci Rep 2015;5:13298.
61. Mansi M, Howley R, Chandratre S, Chen B. Inhibition of ABCG2 transporter by lapatinib enhances 5-aminolevulinic acid-mediated protoporphyrin IX fluorescence and photodynamic therapy response in human glioma cell lines. Biochem Pharmacol 2022;200:115031.
62. Lee HJ, Heo DS, Cho JY, et al. A phase i study of oral paclitaxel with a novel p-glycoprotein inhibitor, HM30181A, in patients with advanced solid cancer. Cancer Res Treat 2014;46:234-42.
63. Choi YH, Yu AM. ABC transporters in multidrug resistance and pharmacokinetics, and strategies for drug development. Curr Pharm Des 2014;20:793-807.
64. Wu CP, Calcagno AM, Ambudkar SV. Reversal of ABC drug transporter-mediated multidrug resistance in cancer cells: evaluation of current strategies. Curr Mol Pharmacol 2008;1:93-105.
65. Shukla S, Chen ZS, Ambudkar SV. Tyrosine kinase inhibitors as modulators of ABC transporter-mediated drug resistance. Drug Resist Updat 2012;15:70-80.
66. Wu CP, Hsiao SH, Huang YH, et al. Sitravatinib Sensitizes ABCB1- and ABCG2-overexpressing multidrug-resistant cancer cells to chemotherapeutic drugs. Cancers 2020;12:195.
67. Rahman I, Liang B, Sajid A, Ambudkar SV, Huang HC. Photodynamic priming modulates cellular ATP levels to overcome P-glycoprotein-mediated drug efflux in chemoresistant triple-negative breast cancer. Photochem Photobiol 2024;Online ahead of print.
68. ClinicalTrials.gov. Photodynamic therapy in treating patients with cancer of the bile duct, gallbladder, or pancreas. Available from: https://clinicaltrials.gov/study/NCT00003923. [Last accessed on 13 Sep 2024].
69. Heinzelmann-Schwarz V, Fedier A, Hornung R, Walt H, Haller U, Fink D. Role of p53 and ATM in photodynamic therapy-induced apoptosis. Lasers Surg Med 2003;33:182-9.
70. Luna MC, Ferrario A, Wong S, Fisher AM, Gomer CJ. Photodynamic therapy-mediated oxidative stress as a molecular switch for the temporal expression of genes ligated to the human heat shock promoter. CancerRes 2000;60:1637-44.
Cite This Article

How to Cite
Vig, S.; Srivastava P.; Rahman I.; Jaranson R.; Dasgupta A.; Perttilä R.; Uusimaa P.; Huang H. C. Screening of photosensitizers-ATP binding cassette (ABC) transporter interactions in vitro. Cancer. Drug. Resist. 2024, 7, 35. http://dx.doi.org/10.20517/cdr.2024.50
Download Citation
Export Citation File:
Type of Import
Tips on Downloading Citation
Citation Manager File Format
Type of Import
Direct Import: When the Direct Import option is selected (the default state), a dialogue box will give you the option to Save or Open the downloaded citation data. Choosing Open will either launch your citation manager or give you a choice of applications with which to use the metadata. The Save option saves the file locally for later use.
Indirect Import: When the Indirect Import option is selected, the metadata is displayed and may be copied and pasted as needed.
About This Article
Copyright
Data & Comments
Data
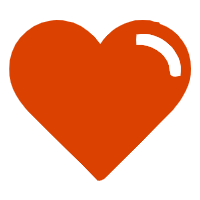
Comments
Comments must be written in English. Spam, offensive content, impersonation, and private information will not be permitted. If any comment is reported and identified as inappropriate content by OAE staff, the comment will be removed without notice. If you have any queries or need any help, please contact us at support@oaepublish.com.