In vitro study of the gel cohesivity and persistence to hyaluronidase degradation of a novel stabilized composition of 26 mg/mL of high molecular weight HA
Abstract
Aim: Hyaluronic acid (HA) is extensively used in injectable skin quality products due to its documented role in skin rejuvenation. The rapid in vivo degradation of HA by the enzyme hyaluronidase necessitates the development of advanced formulations to ensure the efficacy and longevity of the treatments. In this context, a novel 2.6% high molecular weight HA (H-HA)/3.2% sorbitol composition has been introduced, featuring stabilization through hydrogen bonds rather than traditional crosslinking.
Methods: The stabilized composition was evaluated through two in vitro enzymatic degradation tests. In the first test, the efficiency on the gel degradation was followed by rheology and compared with two crosslinked HA products available on the market. In the second test, the effect on the gel structure of a less diluted hyaluronidase dose was followed by rheological and cohesivity measurements.
Results:In vitro study demonstrates that, before its complete degradation into a liquid-like state, the stabilized composition exhibits high elasticity and cohesivity during the enzymatic degradation process, surpassing traditional crosslinked HA products.
Conclusion: The stabilization provided by the sorbitol in the stabilized composition effectively enhances product properties and protects them during gel degradation. These attributes indicate significant potential for improved clinical outcomes in skin quality treatments.
Keywords
INTRODUCTION
The perception of aging is heavily influenced by skin quality. A smooth, firm, and unblemished complexion inherently conveys a sense of youthfulness, while faces marked by wrinkles are often deemed less attractive, less appealing, and less vibrant[1-3]. This perception fuels a growing demand for both corrective and preventive treatments aimed at rejuvenating skin and combatting premature skin aging[4]. Knowledge about the processes and etiology of facial aging has advanced considerably in the last decade[5,6]. The aging process is determined by intrinsic and extrinsic factors such as decreased hormone levels, free radicals, ultraviolet, and even pollution. Consequently, the synthesis of extracellular matrix components is reduced, leading to thinning of epidermis and dermis, as well as weakening of the dermo-epidermal junction. Aging is visually typified by deterioration of skin tone and texture, deflation due to loss of bone and fat, and descent of soft tissues due to loss of muscle tone and skin elasticity. In the past two decades, various injectable formulations called dermal fillers and skin boosters have been marketed to address volume deficiencies and improve skin quality, showing temporary [collagen, hyaluronic acid (HA), calcium hydroxylapatite, poly-l-lactic acid (PLLA), polycaprolactone, etc.] or permanent effects (polymethylmethacrylate)[7-12]. Treatments specifically targeting the extracellular matrix of the dermis to enhance skin texture and tone are commonly categorized as skin boosters or skin quality enhancers (including HA, PLLA, polynucleotides, and exosomes)[2,13].
In the realm of such procedures, the use of HA injectables has been steadily rising, garnering widespread acclaim in the fields of aesthetic medicine and dermatology[1,14-16]. HA is favored for its efficacy in corrective anti-aging and moisturizing treatments, thanks to its ability to safely enhance firmness, reduce fine lines, retain moisture, and regulate skin structure and function. For instance, research demonstrates that HA can enhance skin quality by promoting the spreading and proliferation of fibroblasts, thereby helping to maintain a youthful appearance[17].
HA is a naturally occurring compound with a dynamic turnover rate, exhibiting a half-life of less than
Striking a balance between the two critical factors - longevity and safety - raises important considerations regarding enzymatic degradation. An advanced HA product designed to improve clinical results shall consider hyaluronidase activity to extend the in vivo persistence of the gel while ensuring proper responsiveness to exogenous hyaluronidase injection, thereby allowing for reversible treatment in case of complications. This significant challenge necessitates innovative strategies. One such approach involves the use of crosslinked HA, especially with 1, 4-butanediol diglycidyl ether (BDDE) crosslinker. These crosslinked structures create a barrier against enzymatic degradation, resulting in improved durability and viscoelasticity[31]. Increasing the degree of HA crosslinking reduces the propensity to degradation[32-36]; however, this method alters the HA from its natural form, i.e., the linear hyaluronan, which is not chemically modified. Therefore, manufacturers shall identify an optimal balance to develop a crosslinked HA gel that achieves the desired longevity and biophysical properties while remaining as close as possible to the natural hyaluronan, thus maximizing safety.
Recent research advancements have introduced a novel stabilized 2.6% high molecular weight hyaluronic acid (H-HA)/3.2% sorbitol composition, which is aimed at improving skin quality by enhancing attributes such as elasticity and hydration. This formulation, designed to be injected in subcutaneous tissues, contains 26 mg/mL of H-HA stabilized with 32 mg/mL of sorbitol, a highly safe molecule, in a phosphate buffer solution. Unlike crosslinked products that utilize high-energy chemical covalent bonds between the crosslinker and the reactive ends of HA, this new formulation relies on a high content of low-energy hydrogen bonds between sorbitol and HA, without any chemical modifications to the HA [Figure 1].
Figure 1. Schematic representation of HA structures obtained through (A) Crosslinking of HA via covalent bonds (cases of VYC-12 and NASHA-20 products) and (B) Stabilization of HA via a dense matrix of hydrogen bonds for the 2.6% H-HA/3.2% sorbitol product. HA: Hyaluronic acid.
In other words, the HA chains are not linked by covalent bonds generated by a crosslinker such as BDDE, but by hydrogen bonds, which are intermolecular interactions of low energy that can be easily broken and restored depending on the mechanical actions applied to the product. This characteristic is a key advantage, allowing the product to exhibit a more natural behavior in the skin and resulting in a highly flexible gel that behaves like the surrounding tissues.
A recent study reported the unique biophysical properties, appropriate distribution, and high tolerance of this stabilized 2.6% H-HA/3.2% sorbitol composition in adipose tissue, along with its ability to efficiently improve skin firmness[37]. Another study demonstrates this formulation’s efficacy in improving biological markers of skin quality in the dermis after injection into the superficial adipose tissue, providing evidence for the biostimulation of endogenous HA, elastin, collagens, and fibrillin production[38]. This article aims to evaluate the gel cohesivity and persistence of the stabilized 2.6% H-HA/3.2% sorbitol composition, specifically its resistance to degradation during hyaluronidase activity. This investigation involves various in vitro tests. Additionally, the properties and performance of this novel product are also compared to those of leading commercially available crosslinked HA products used as skin boosters in aesthetic medicine.
METHODS
Materials
The current study examines the following three injectable products:
Stabilized 2.6% H-HA/3.2% sorbitol composition (Kylane Laboratoires, Switzerland) contains 26 mg/mL of H-HA stabilized with 32 mg/mL of sorbitol. This skin booster is indicated for enhancing skin quality attributes such as elasticity and hydration, as well as for correcting small skin depressions.
Juvéderm Volite® (VYC-12, Allergan, France), a crosslinked HA gel manufactured using Vycross technology. It contains 12 mg/mL of crosslinked HA and 3 mg/mL of lidocaine, and is indicated for hydrating the skin, improving skin quality, and correcting superficial cutaneous depressions, such as fine lines.
Restylane® Skinboosters™ Vital Lidocaine (NASHA-20, Galderma, Sweden) is a crosslinked HA gel manufactured using NASHA™ technology. It contains 20 mg/mL of crosslinked HA and 3 mg/mL of lidocaine, and is indicated for improving skin hydration, texture, and elasticity.
Rheological measurements
In this study, rheological measurements were conducted using 1.0 g of each sample placed on a TA Instrument HR10 rheometer at 37 °C (Peltier plate). The rheometer was equipped with a cone-plate aluminum geometry of 40 mm diameter, 2° cone angle, and a 50-μm gap between the cone and the plate.
The elastic modulus G’ was recorded in oscillation mode at a strain of 1.0% within the linear viscoelastic region and at a physiological oscillation frequency of 1 Hz. Each measurement was performed in triplicate, and the final mean value is reported in this study.
Cohesivity measurements
Cohesivity tests were conducted following the method described by Sundaram et al.[39]. Each sample’s cohesivity was ranked at 15, 70, and 95 s using the Gavard-Sundaram Cohesivity Scale (GSCS), where scores range from 1 (fully dispersed) to 5 (fully cohesive). Evaluations were performed separately by three experts, who were trained in the cohesivity ranking method based on the GSCS. The final mean value is reported in this study.
Enzymatic degradation
Two tests were conducted to quantitively study the physicochemical changes in the properties of HA gel during enzymatic degradation by hyaluronidase:
Test 1
The purpose of Test 1 is to simulate the conditions of a hyaluronidase injection procedure performed by a healthcare professional to address a complication (i.e., adverse event) requiring the degradation of HA gel in skin tissues. This test necessitates a significant dilution of the HA gel to enhance its degradation in the skin.
In this study, a fresh hyaluronidase solution at a concentration of 60 U/mL was prepared by dissolving a lyophilized powder of hyaluronidase sourced from bovine testes (Sigma Aldrich, 748 U/mg) in a phosphate buffer solution at pH 7.2. The HA product and the enzymatic solution were incubated separately at 37 °C in an oven for 12 min. Following this, equivolumes of the HA product and the enzymatic solution were mixed for 1 min. Immediately after mixing, 1.0 g of the blend was placed on the rheometer to monitor the gel elastic modulus G’ in real time, as described in the “rheological measurements” section. Measurements were taken regularly until the G’ reached a limit of 10 Pa, indicating complete degradation to a liquid-like state. Notably, the first exploitable experimental point was observed at 1.5 min after the initial contact between the hyaluronidase solution and the HA product (t0). To accurately assess the kinetics of degradation during the initial moments, the G’ value at t0 was determined from a blank test conducted for each tested HA product (i.e., equivolume mixture of the HA gel and phosphate buffer solution), with the only difference being the absence of hyaluronidase in the phosphate buffer solution.
The hyaluronidase Test 1 was conducted on the stabilized 2.6% H-HA/3.2% sorbitol composition, VYC-12 and NASHA-20 products.
Test 2
The aim of Test 2 is to simulate the conditions for hyaluronidase activity with limited dilution of the HA gel. In contrast to hyaluronidase Test 1, Test 2 does not require significant dilution with the phosphate buffer solution containing hyaluronidase; instead, it aims to restrict gel dilution to better assess the impact of hyaluronidase degradation on HA while observing the visual effects on product structure through cohesivity assessment.
For Test 2, a more concentrated enzymatic solution (1,500 U/mL) was prepared by dissolving a lyophilized hyaluronidase powder from bovine testes (Sigma Aldrich, 748 U/mg) in a phosphate buffer solution at pH 7.2. The HA product to be tested, colored with 0.1% toluidine blue powder (Sigma Aldrich), and the enzymatic solution were incubated separately at 37 °C in an oven for 12 min. The hyaluronidase solution was then added at 4% v/v to the tested gel, resulting in an enzymatic concentration of 60 U/g of gel. This mixture was homogenized for 1 min before a portion was placed on the rheometer to record the elastic modulus G’ as a function of time, following the procedure described in the “rheological measurements” section. Meanwhile, the remaining mixture was returned to the oven at 37 °C, and 1.0 g of this blend was sampled at different time points for the cohesivity test as outlined in the “cohesivity measurements” section.
This hyaluronidase Test 2 was conducted exclusively with the stabilized 2.6% H-HA/3.2% sorbitol composition.
Statistical analysis
Quantitative variables are expressed as means ± standard deviations (SD) based on n = 3 replicates per group. The 95% Confidence Interval (95%CI) is indicated in brackets.
Inferential statistical comparisons of the means are conducted pairwise using a parametric Student’s t-test. The initial data are unpaired, normally distributed (Shapiro-Wilk test), and exhibit equal variances (Levene’s test). The Student’s t-test provides a P-value, representing the probability that the two populations of results are significantly different. A Bonferroni correction is applied to the significance level α, set at 0.017. The differences are categorized as follows:
- Not significant if P-value ≥ 0.017 (ns);
- Slightly significant if P < 0.017(*);
- Significant if P < 0.0033 (**);
- Highly significant if P < 0.00033 (***).
RESULTS
Measurements of cohesivity
The cohesivity results for the novel stabilized 2.6% H-HA/3.2% sorbitol composition are very high, reaching the maximum on the GSCS, as illustrated in Table 1. After 95 s, the GSCS score for this composition remains at 5.0 ± 0.0 (fully cohesive). This new product exhibits much higher cohesivity compared to the two crosslinked skin quality products used for comparison in this study. Notably, after just 15 s, the most cohesive crosslinked product NASHA-20 scores 4.0 ± 0.0 (mostly cohesive). After 95 s, the cohesivity scores for NASHA-20 and VYC-12 decrease to 2.7 ± 0.6 and 1.0 ± 0.0, respectively.
Images and cohesivity scores for the three tested HA-based products (means ± standard deviations for n = 3 replicates per group)
Tested composition | Images and cohesivity scores at different time points | ||
15 s | 70 s | 95 s | |
2.6% H-HA/3.2% sorbitol | ![]() | ![]() | ![]() |
5.0 ± 0.0 | 5.0 ± 0.0 | 5.0. ± 0.0 | |
VYC-12 | ![]() | ![]() | ![]() |
1.7 ± 0.6 | 1.0 ± 0.0 | 1.0 ± 0.0 | |
NASHA-20 | ![]() | ![]() | ![]() |
4.0 ± 0.0 | 2.7 ± 0.6 | 2.7± 0.6 |
Measurement of elastic modulus G’
On the other hand, as shown in Table 2, the elastic modulus G’ of the stabilized 2.6% H-HA/3.2% sorbitol composition is 523 Pa [SD = 13; 95%CI: (508, 539)], significantly exceeding the values of conventional BDDE-crosslinked products: NASHA-20 [376 Pa; SD = 33; 95%CI: (339, 414); P-value = 0.021] and VYC-12 [158 Pa; SD = 16; 95%CI: (140, 177); P-value = 10-5] products.
Elastic modulus G’ of three tested HA-based products (means ± standard deviations for n = 3 replicates per group)
Tested composition | Values at 1 Hz |
Elastic modulus G’ (Pa) | |
2.6% H-HA/3.2% sorbitol | 523 ± 13 |
VYC-12 | 158 ± 16 |
NASHA-20 | 376 ± 33 |
Monitoring of the enzymatic degradation of HA gels in highly diluted conditions (Test 1)
These outstanding initial properties of HA gels were subsequently assessed in terms of enzymatic degradation. The hyaluronidase Test 1, presented in Figure 2, simulates the enzymatic degradation relevant to treating adverse events. The three rheological curves in Figure 2A represent typical enzymatic degradation patterns for HA products. The degradation kinetics start rapidly in the first seconds and then gradually decelerate until they reach a point deemed negligible. Interestingly, this limit in G’ appears consistent across all three products. Once the G’ value falls below 10 Pa, no further changes in the elastic modulus are observed, indicating complete degradation and a transition to a liquid-like state. In this context, the stabilized 2.6% H-HA/3.2% sorbitol composition demonstrates greater resistance to hyaluronidase degradation than the two crosslinked formulations, VYC-12 and NASHA-20. However, the time required for complete degradation of the gel is less than 40 min. Interestingly, the data in Figure 2B reveal that the stabilized 2.6% H-HA/3.2% sorbitol composition degrades significantly more slowly [39.2 min; SD = 2.1; 95%CI: (36.8, 41.5)] compared to the two leading crosslinked skin quality products used for comparison. This observation cannot be explained solely by HA concentration, as the less concentrated VYC-12 [21.8 min; SD = 8.6; 95%CI: (12.1, 31.6)] shows better resistance to enzymatic degradation than NASHA-20 [14.6 min; SD = 3.0; 95%CI: (11.2, 18.0)], although the difference is not statistically significant.
Figure 2. ns: Not significant difference (P ≥ 0.017). ***highly significant difference (P < 0.00033); *slightly significant difference (P < 0.017). (A) Representative curves of elastic modulus G’ (Pa) as a function of time during enzymatic treatment with 1:1 v/v of 60 U of hyaluronidase per gram of gel; (B) Time required for complete enzymatic degradation (G’ < 10 Pa) for the three tested skin quality treatments. Assessments of normality and homogeneity of variances for the datasets are available in Supplementary Table 2. H-HA: High molecular weight hyaluronic acid.
Monitoring of the enzymatic degradation of 2.6% H-HA/3.2% sorbitol composition under concentrated conditions (Test 2)
The hyaluronidase Test 2, presented in Figure 3, provides insights into the mechanisms involved in the enzymatic degradation of the stabilized 2.6% H-HA/3.2% sorbitol composition. The data show a positive correlation between the elastic modulus G’ and the cohesivity scores. As the stabilized gel undergoes gradual degradation, it progressively loses its rheological properties and cohesivity. Notably, both the elastic modulus G’ and the cohesivity are remarkably preserved throughout the degradation process. During the later stages of enzymatic degradation (i.e., from 8 min to 50 min), the cohesivity of the stabilized 2.6% H-HA/3.2% sorbitol composition remains comparable to that of the two crosslinked VYC-12 and NASHA-20 products, as illustrated in Table 1. Additionally, the cohesivity data further confirm that the degradation of the stabilized 2.6% H-HA/3.2% sorbitol composition by hyaluronidase leads to a complete breakdown of the composition. This is characterized in Figure 3 by a cohesivity level considered negligible at the end of the hyaluronidase Test 2. After 50 min of reaction between the hyaluronidase and the stabilized 2.6% H-HA/3.2% sorbitol composition, the product appears to be completely dissolved, transitioning from a gel or gel fragments to a liquid state devoid of any internal structure and strength.
Figure 3. The grey line represents the elastic modulus G’ (Pa) as a function of time for the stabilized 2.6% H-HA/3.2% sorbitol composition degraded by enzymatic treatment with 4% v/v of 60 U of hyaluronidase per gram of gel (means ± standard deviations for n = 3 replicates); The blue line indicates the cohesivity scores at 95 s for the same stabilized composition, sampled at different degradation times (means ± standard deviations for n = 3 replicates). The figure caption displays images from the cohesivity tests at
DISCUSSION
HA polymers consist of long chains of repeating disaccharide units, specifically glucuronic acid and N-acetylglucosamine, which are linked by glycosidic bonds. This linear, hydroxyl-rich structure imparts HA with exceptional viscoelastic properties and water-binding capacity. In most injectable skin-quality HA-based products, such as Juvéderm Volite® (VYC-12, Allergan, France) and Restylane® Skinboosters™ Vital Lidocaine (NASHA-20, Galderma, Sweden), HA molecules are crosslinked using 1,4- BDDE. The epoxide groups in BDDE preferentially react with the most accessible primary alcohol groups in the HA backbone, forming covalent ether bonds. The degree of modification (MoD) in crosslinked products typically ranges from 1% to 10%[39], which is sufficient to create a rigid three-dimensional structure that reduces the relative mobility of the HA chains. This scaffolding enhances the elasticity G’ of the HA gel and acts as a barrier, limiting the accessibility of reactive molecules, such as hyaluronidase or reactive oxygen species (ROS), which can degrade the gel. Consequently, the stability and longevity of crosslinked HA within the skin are increased[31].
The internal strength of HA gels is not solely dependent on crosslinking; rather, it results from a careful balance among HA concentration, degree of crosslinking, crosslinking technology, and physicochemical MoD[40]. Each of these factors could be predominant over the others depending on the specific gels being studied[41-43].
In contrast to traditional crosslinked HA products that rely on a few strong covalent junctions, the novel stabilized 2.6% H-HA/3.2% sorbitol composition features a distinct HA structure. This structure is achieved through a specific formulation and process that creates multiple hydrogen bonds within the gel matrix, as illustrated in Figure 1.
Due to the weak nature of hydrogen bonds are weak, one might intuitively conclude that the generated network is more sensitive to intense external mechanical forces than a crosslinked product. In fact, the strength of a typical hydrogen bond is approximately 5% that of an average covalent bond[44]. However, from a general chemistry perspective, hydrogen bonding between molecules is limited only by the number of hydrogen atoms covalently bonded to elements such as oxygen or nitrogen. The presence of more hydrogen bonds increases the overall strength, significantly contributing to intermolecular interactions, which lead to remarkable properties[45]. In the stabilized 2.6% H-HA/3.2% sorbitol composition, the arrangement of sorbitol and HA is particularly advantageous, as shown in Figure 1. Both molecules contain numerous hydroxyl groups along their structure. As sorbitol is a small molecule (C6 sugar), it can easily fit between the long chains of H-HA, maximizing mutual attraction and reinforcing the resulting network[46] by forming a highly dense matrix of hydrogen bonds within the gel.
The results presented in Tables 1 and 2 indicate that HA/sorbitol stabilization significantly enhances cohesivity and elasticity G’. These unique features, previously established by Gavard et al.[37] and further supported in this study with consistent additional data, are crucial for offering new advantages and improving clinical outcomes in skin quality treatment, positioning them as a new category of advanced skin boosters. The high concentration of H-HA, along with its exceptional cohesivity and elasticity G’, contributes favorably to tissue interaction in the treated area, thereby enhancing mechano-stimulation and promoting better tissue regeneration.
In investigating the performance profile of injectable HA products for skin quality treatments, evaluating the persistence of HA against hyaluronidase degradation is a crucial factor. This article presents a comprehensive in vitro study of this enzymatic degradation, supported by rheological and cohesivity evidence. Literature reports on the dose, volume, and administration techniques for exogenous hyaluronidase injections by healthcare professionals to manage complications (i.e., adverse events). Research has examined a wide range of doses, from 1.5 up to over 1,000 U, with a consensus indicating that around 60 U of hyaluronidase can effectively degrade 1.0 g of most HA-based products[47].
In the present study, hyaluronidase Test 1 examines enzymatic degradation using a concentration of 60 U/g of gel. Equivolumes of the HA product and the enzymatic solution are mixed to achieve this concentration. The significant dilution effect of the HA gel reflects the conditions typically encountered when treating adverse events that require the degradation of HA gel within skin tissues. Practitioners used to inject large volumes of hyaluronidase solution into the gel to facilitate gel resorption and enhance enzyme diffusion. In such cases, a complete and fast degradation is anticipated, ideally occurring within 1 h, as hyaluronidase activity could be only partially maintained in vivo during this time[48]. Additionally, in hyaluronidase Test 1, the rheological monitoring of the elastic modulus G’ as a function of time was conducted to assess the persistence of the gel structure for each tested product.
Whatever the product tested, the time required for complete degradation of the gel is under 40 min, as presented in Figure 2. This aligns with practitioners’ need to activate the degradation of the HA gel in less than 1 h during clinical treatments for complications. However, the data surprisingly indicate that the stabilized 2.6% H-HA/3.2% sorbitol composition degrades significantly more slowly than the two leading crosslinked skin quality products used for comparison.
These results can be considered unconventional because the crosslinking of HA is widely recognized as the most effective method for enhancing HA’s resistance to hyaluronidase activity. Higher concentrations of HA and a greater degree of crosslinking are associated with increased resistance to enzymatic degradation[19,49,50], although crosslinking technology seems to be the predominant factor[50]. NASHA-based products are reported to be among the most sensitive to hyaluronidase, whereas HA products manufactured with Vycross technology exhibit greater resistance to degradation. Therefore, the results of this article regarding HA products NASHA-20 (Galderma) and VYC-12 (Allergan), used for comparison with the stabilized 2.6% H-HA/3.2% sorbitol composition, align with findings from other published studies[49-51].
This better resistance of the stabilized 2.6% H-HA/3.2% sorbitol composition to hyaluronidase degradation can be explained by the unique stabilized structure of the HA gel. This structure is strongly densified by the high density of hydrogen bonds formed by the sorbitol molecules within the viscoelastic gel matrix, as illustrated in Figure 1. The numerous hydrogen bonds in this configuration impede the action of hyaluronidase, effectively serving as a natural shield that protects the HA gel from enzymatic degradation.
To better understand the kinetics of hyaluronidase action on this structure, the hyaluronidase Test 2 facilitates the comprehensive mapping of the structure and internal strength of the stabilized 2.6% H-HA/3.2% sorbitol composition throughout enzymatic degradation, as presented in Figure 3. The dilution factor of the gel is significantly lower than in the hyaluronidase Test 1, while the ratio of hyaluronidase content to HA remains the same. This approach allows for representative cohesivity measurements throughout the experiment, enabling visual observation of degradation’s impact on the gel’s structure. With this analysis of Test 2, the loss of elastic modulus G’ while retaining cohesivity may seem paradoxical, but it can be explained by the rapid rearrangement of hydrogen bonds within the HA matrix. Once hyaluronidase reaches its target site and breaks HA, a new accessible hydroxyl group is generated, allowing for the formation of a new hydrogen bond with sorbitol. This rearrangement occurs rapidly, typically within a few nanoseconds[52,53], conferring a dynamic “self-healing” characteristic to the product. Specifically, this phenomenon compensates for the loss of structural cohesivity due to HA network degradation by creating an immediate secondary network [Figure 4]. However, as HA molecules are irreversibly cut, their overall length decreases, leading to increased mobility among the chains. Consequently, the newly formed network suffers from a lack of macromolecular organization, resulting in a decrease in elastic modulus G’[54,55]. Internal cohesion is ultimately lost when low molecular weight HA predominates in the gel, causing molecular chains to become so mobile that sorbitol can no longer effectively serve as internal cement.
Figure 4. Schematic representation of the stabilized 2.6% H-HA/3.2% sorbitol composition at different states of hyaluronidase degradation (glycosidic bond attacks), illustrating the preservation of cohesivity due to the dense network of hydrogen bonds within the gel structure. H-HA: High molecular weight hyaluronic acid.
The cohesivity and resistance to hyaluronidase degradation observed in the stabilized 2.6% H-HA/3.2% sorbitol composition are significant features that enable prolonged performance in skin tissues through mechano-stimulation. This process may enhance the skin’s endogenous production of key components such as HA, elastin, collagen, and fibrillin, as previously reported in the literature[38].
The findings presented in this study shall be interpreted with consideration of its inherent limitations. Using triplicate datasets introduced several challenges, including sensitivity to outliers, imprecise variability estimation, reduced accuracy in confidence intervals, and limited generalizability. These factors may result in undetected small differences between products. However, the confirmed normal distributions and high statistical power significantly mitigated many common biases associated with triplicates.
Only a limited selection of available skin quality boosters from the broader market was investigated. Therefore, our results could be strengthened through comparative studies with other skin quality enhancers available.
On the other hand, while in vitro assays provide a controlled environment for assessing resistance to hyaluronidase degradation, they do not fully replicate the complexity of living tissues. Future in vivo studies and clinical trials are needed to address this limitation, offering more accurate insights into the treatment’s longevity, safety profile, and effects on skin hydration, elasticity, and overall quality over time.
In conclusion, the findings of this study demonstrate that the stabilized 2.6% H-HA/3.2% sorbitol composition exhibits unique elasticity (G’) properties and cohesivity, which are well-preserved during enzymatic degradation by hyaluronidase. The stability of this novel composition is significantly higher than that of the crosslinked HA VYC-12 and NASHA-20 products. This indicates that the incorporation of sorbitol into the H-HA effectively enhances product properties and protects them during degradation in skin tissues. This sustained high elasticity G’ and cohesivity during the in vitro degradation of the 2.6% H-HA/3.2% sorbitol composition should be regarded as a key differentiating factor that enhances product performance in skin quality treatments. This ability strengthens the potential of this advanced stabilized HA product to boost the mechano-stimulation of skin tissues, improving skin structure and texture, hydration and elasticity. Importantly, it also allows for a reversible treatment option with hyaluronidase in case of complications.
With its composition and features, the stabilized 2.6% H-HA/3.2% sorbitol product represents a promising new category of skin boosters in anti-aging and regenerative treatments.
DECLARATIONS
Authors’ contributions
Design of the concept, the collection of experimental data, and the writing of the article: Finke A, Bon Bétemps J, Gavard Molliard S
Availability of data and materials
All supporting data are available in the full text or as Supplementary Materials.
Financial support and sponsorship
Kylane Laboratoires SA provided logistical and financial support for the conduct of this study.
Conflicts of interest
All authors are employed by Kylane Laboratoires SA.
Ethical approval and consent to participate
Not applicable.
Consent for publication
Not applicable.
Copyright
© The Author(s) 2024.
Supplementary Materials
REFERENCES
1. Tatarunaite E, Playle R, Hood K, Shaw W, Richmond S. Facial attractiveness: a longitudinal study. Am J Orthod Dentofacial Orthop 2005;127:676-82.
2. Goldie K, Kerscher M, Fabi SG, et al. Skin quality - a holistic 360° view: consensus results. Clin Cosmet Investig Dermatol 2021;14:643-54.
3. Ebner NC. Age of face matters: age-group differences in ratings of young and old faces. Behav Res Methods 2008;40:130-6.
4. Anti-aging products market size, share & trends analysis report by product (facial cream & lotion, eye cream & lotion), by distribution channel (hypermarket & supermarket, specialty store), and segment forecasts, 2021-2028. Available from: https://www.grandviewresearch.com/industry-analysis/anti-aging-products-market/request/rs2 [Last accessed on 21 Oct 2024].
5. Zargaran D, Zoller F, Zargaran A, Weyrich T, Mosahebi A. Facial skin ageing: key concepts and overview of processes. Int J Cosmet Sci 2022;44:414-20.
6. Swift A, Liew S, Weinkle S, Garcia JK, Silberberg MB. The facial aging process from the “Inside Out”. Aesthet Surg J 2021;41:1107-19.
7. Salvatore L, Natali ML, Brunetti C, Sannino A, Gallo N. An update on the clinical efficacy and safety of collagen injectables for aesthetic and regenerative medicine applications. Polymers (Basel) 2023;15:1020.
8. Amiri M, Meçani R, Niehot CD, et al. Skin regeneration-related mechanisms of calcium hydroxylapatite (CaHA): a systematic review. Front Med (Lausanne) 2023;10:1195934.
9. Corduff N, Juniarti L, Lim TS, et al. Current practices in hyaluronic acid dermal filler treatment in asia pacific and practical approaches to achieving safe and natural-looking results. Clin Cosmet Investig Dermatol 2022;15:1213-23.
10. Ao YJ, Yi Y, Wu GH. Application of PLLA (poly-l-lactic acid) for rejuvenation and reproduction of facial cutaneous tissue in aesthetics: a review. Medicine (Baltimore) 2024;103:e37506.
11. Christen MO, Vercesi F. Polycaprolactone: how a well-known and futuristic polymer has become an innovative collagen-stimulator in esthetics. Clin Cosmet Investig Dermatol 2020;13:31-48.
12. Al-Ghanim K, Richards R, Cohen S. A practical guide to selecting facial fillers. J Cosmet Dermatol 2023;22:3232-6.
13. Yi KH, Winayanuwattikun W, Kim SY, et al. Skin boosters: definitions and varied classifications. Skin Res Technol 2024;30:e13627.
14. Cassuto D, Bellia G, Schiraldi C. An overview of soft tissue fillers for cosmetic dermatology: from filling to regenerative medicine. Clin Cosmet Investig Dermatol 2021;14:1857-66.
15. Kleine-Börger L, Meyer R, Kalies A, Kerscher M. Approach to differentiate between hyaluronic acid skin quality boosters and fillers based on their physicochemical properties. J Cosmet Dermatol 2022;21:149-57.
16. Ghatge AS, Ghatge SB. The effectiveness of injectable hyaluronic acid in the improvement of the facial skin quality: a systematic review. Clin Cosmet Investig Dermatol 2023;16:891-9.
17. Kutty JK, Cho E, Soo Lee J, Vyavahare NR, Webb K. The effect of hyaluronic acid incorporation on fibroblast spreading and proliferation within PEG-diacrylate based semi-interpenetrating networks. Biomaterials 2007;28:4928-38.
18. Laurent UB, Dahl LB, Reed RK. Catabolism of hyaluronan in rabbit skin takes place locally, in lymph nodes and liver. Exp Physiol 1991;76:695-703.
19. Fraser JR, Laurent TC, Laurent UB. Hyaluronan: its nature, distribution, functions and turnover. J Intern Med 1997;242:27-33.
20. Paap MK, Silkiss RZ. The interaction between hyaluronidase and hyaluronic acid gel fillers - a review of the literature and comparative analysis. Plast Aesthet Res 2020;7:36.
21. Ludowieg J, Vennesland B, Dorfman A. The mechanism of action of hyalurinidase. Biol Chem 1961;236:333-9. Available from:
22. Berdiaki A, Neagu M, Spyridaki I, Kuskov A, Perez S, Nikitovic D. Hyaluronan and reactive oxygen species signaling-novel cues from the matrix? Antioxidants (Basel) 2023;12:824.
23. Soltés L, Mendichi R, Kogan G, Schiller J, Stankovska M, Arnhold J. Degradative action of reactive oxygen species on hyaluronan. Biomacromolecules 2006;7:659-68.
24. Beleznay K, Humphrey S, Carruthers JD, Carruthers A. Vascular compromise from soft tissue augmentation: experience with 12 cases and recommendations for optimal outcomes. J Clin Aesthet Dermatol 2014;7:37-43.
25. Ozturk CN, Li Y, Tung R, Parker L, Piliang MP, Zins JE. Complications following injection of soft-tissue fillers. Aesthet Surg J 2013;33:862-77.
26. Mehta P, Kaplan JB, Zhang-nunes S. Ischemic complications of dermal fillers. Plast Aesthet Res 2022;9:57.
28. Chatrath V, Banerjee PS, Goodman GJ, Rahman E. Soft-tissue filler-associated blindness: a systematic review of case reports and case series. Plast Reconstr Surg Glob Open 2019;7:e2173.
29. Diwan Z, Trikha S, Etemad-Shahidi S, Parrish N, Rennie C. Evaluation of current literature on complications secondary to lip augmentation following dermal filler injection. J Clin Aesthet Dermatol 2023;16:26-33.
30. Oranges CM, Brucato D, Schaefer DJ, Kalbermatten DF, Harder Y. Complications of nonpermanent facial fillers: a systematic review. Plast Reconstr Surg Glob Open 2021;9:e3851.
31. Falcone SJ, Berg RA. Crosslinked hyaluronic acid dermal fillers: a comparison of rheological properties. J Biomed Mater Res A 2008;87:264-71.
32. Rao V, Chi S, Woodward J. Reversing facial fillers: interactions between hyaluronidase and commercially available hyaluronic-acid based fillers. J Drugs Dermatol 2014:13;1053-6.
33. Jones D, Tezel A, Borrell M. In Vitro resistance to degradation of hyaluronic acid dermal fillers by ovine testicular hyaluronidase. Dermatol Surg 2010;36:804-9.
34. Sall I, Férard G. Comparison of the sensitivity of 11 crosslinked hyaluronic acid gels to bovine testis hyaluronidase. Polym Degrad Stab 2007;92:915-9.
35. Cavallini M, Papagni M, Trocchi, G. Sensitivity of hyaluronic acid fillers to hyaluronidase: an in vitro analysis. J Clin Exp Dermatol Res 2020;11:1-6. Available from:
36. Juhász MLW, Levin MK, Marmur ES. The kinetics of reversible hyaluronic acid filler injection treated with hyaluronidase. Dermatol Surg 2017;43:841-7.
37. Molliard S, Bon Bétemps J, Hadjab B, Ghazal A, Badi M, Cerrano M. Stabilized composition of 26 mg/mL of high molecular weight HA for subcutaneous injection to improve skin quality. Plast Aesthet Res 2022;9:52.
38. Bétemps J, Gavard Molliard S, Hadjab B, Badi M, Ghazal A, Cerrano M. Study of biological markers in skin quality treatment by subcutaneous injection of a stabilized composition of 26 mg/mL of high molecular weight HA. Plast Aesthet Res 2022;9:59.
39. Sundaram H, Rohrich RJ, Liew S, et al. Cohesivity of hyaluronic acid fillers: development and clinical implications of a novel assay, pilot validation with a five-point grading scale, and evaluation of six U.S. food and drug administration-approved fillers. Plast Reconstr Surg 2015;136:678-86.
40. Edsman K, Nord LI, Ohrlund A, Lärkner H, Kenne AH. Gel properties of hyaluronic acid dermal fillers. Dermatol Surg 2012;38:1170-9.
41. Nogami E, Watanabe I, Hoshi H, et al. D-sorbitol can keep the viscosity of dispersive ophthalmic viscosurgical device at room temperature for long term. Sci Rep 2019;9:16815.
42. Pierre S, Liew S, Bernardin A. Basics of dermal filler rheology. Dermatol Surg 2015;41 Suppl 1:S120-6.
43. Zerbinati N, Sommatis S, Maccario C, et al. Toward physicochemical and rheological characterization of different injectable hyaluronic acid dermal fillers cross-linked with polyethylene glycol diglycidyl ether. Polymers (Basel) 2021;13:948.
44. Frey PA. Low barrier hydrogen bonds. In: Lennarz WJ, Lane MD, editors. Encyclopedia of Biological Chemistry. Elsevier; 2004. pp. 594-98
45. Nelson DL, Cox MM. Lehninger principles of biochemistry. 6th ed. W. H. Freeman and Company: New York, USA; 2012. pp. 47-56. Available from: https://books.google.com/books?hl=en&lr=&id=5Ek9J4p3NfkC&oi=fnd&pg=PP2&dq=Nelson+DL,+Cox+MM.+Lehninger+Principles+of+Biochemistry.+6th+ed.+W.+H.+Freeman+and+Company:+New+York,+USA%3B+2012.+pp.+47-56.&ots=ZBDJKBptqI&sig=H4WxaL7fgSftdc7c5_RI9eX_IN0#v=onepage. [Last accessed on 21 Oct 2024].
46. Tezel A, Fredrickson GH. The science of hyaluronic acid dermal fillers. J Cosmet Laser Ther 2008;10:35-42.
47. King M, Convery C, Davies E. This month’s guideline: the use of hyaluronidase in aesthetic practice (v2.4). J Clin Aesthet Dermatol 2018;11:E61-8.
48. Kim HJ, Kwon SB, Whang KU, Lee JS, Park YL, Lee SY. The duration of hyaluronidase and optimal timing of hyaluronic acid (HA) filler reinjection after hyaluronidase injection. J Cosmet Laser Ther 2018;20:52-7.
49. Park KE, Mehta P, Kherani F, et al. Response of 21 hyaluronic acid fillers to recombinant human hyaluronidase. Plast Reconstr Surg Glob Open 2023;11:e5457.
50. Ryu C, Lu JE, Zhang-Nunes S. Response of twelve different hyaluronic acid gels to varying doses of recombinant human hyaluronidase. J Plast Reconstr Aesthet Surg 2021;74:881-9.
51. Faivre J, Wu K, Gallet M, Sparrow J, Bourdon F, Gallagher CJ. Comparison of hyaluronidase-mediated degradation kinetics of commercially available hyaluronic acid fillers in vitro. Aesthet Surg J 2024;44:NP402-10.
52. Kajimoto S, Seong NH, Fukumura H, Dlott DD. Picosecond dynamics of hydrogen bond rearrangements during phase separation of a triethylamine and water mixture. Photochem Photobiol Sci 2014;13:891-7.
53. Tay KA, Bresme F. Kinetics of hydrogen-bond rearrangements in bulk water. Phys Chem Chem Phys 2009;11:409-15.
54. Snetkov P, Zakharova K, Morozkina S, Olekhnovich R, Uspenskaya M. Hyaluronic acid: the influence of molecular weight on structural, physical, physico-chemical, and degradable properties of biopolymer. Polymers (Basel) 2020;12:1800.
Cite This Article
How to Cite
Finke, A.; Bon Bétemps J.; Gavard Molliard, S. In vitro study of the gel cohesivity and persistence to hyaluronidase degradation of a novel stabilized composition of 26 mg/mL of high molecular weight HA. Plast. Aesthet. Res. 2024, 11, 51. http://dx.doi.org/10.20517/2347-9264.2024.88
Download Citation
Export Citation File:
Type of Import
Tips on Downloading Citation
Citation Manager File Format
Type of Import
Direct Import: When the Direct Import option is selected (the default state), a dialogue box will give you the option to Save or Open the downloaded citation data. Choosing Open will either launch your citation manager or give you a choice of applications with which to use the metadata. The Save option saves the file locally for later use.
Indirect Import: When the Indirect Import option is selected, the metadata is displayed and may be copied and pasted as needed.
About This Article
Special Issue
Copyright
Data & Comments
Data
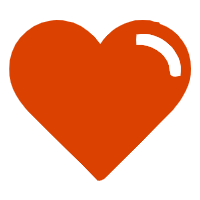
Comments
Comments must be written in English. Spam, offensive content, impersonation, and private information will not be permitted. If any comment is reported and identified as inappropriate content by OAE staff, the comment will be removed without notice. If you have any queries or need any help, please contact us at support@oaepublish.com.