Respiratory abnormalities in Dravet syndrome: insights from a case report and literature review
Abstract
This study investigates the respiratory abnormalities in Dravet Syndrome (DS) through a detailed case report and a narrative literature review. We report the case of a 4-year-old girl, harboring a de novo SCN1A mutation, who exhibited recurrent hypoxemic respiratory failure during febrile infections, not constantly associated with seizures or status epilepticus. In addition, a comprehensive review, including 21 cases, highlights the multifaceted nature of respiratory complications in DS. Notably, severe episodes could occur during common respiratory febrile infections, suggesting an association between DS and increased respiratory risk during febrile illnesses. In fact, the severity and persistence of hypoxemia, even during mechanical ventilation, suggest potential subacute respiratory impairment following seizures and/or infections. The proposed hypothesis of autonomic dysregulation could provide a framework for understanding the intricate relationship between neurological, cardiac, and respiratory functions in DS. This study emphasizes the need for tailored clinical management during febrile illnesses, considering the potential for apnea-related arrhythmias and challenging-to-treat hypoxemia. Further research, including longitudinal studies and advanced physiological monitoring, is crucial for refining our understanding and informing targeted interventions for respiratory abnormalities in DS.
Keywords
INTRODUCTION
Individuals with Dravet Syndrome (DS) face a notable susceptibility to Sudden Unexpected Death in Epilepsy (SUDEP), with an elevated risk of premature mortality affecting approximately 4%-12% of children[1], a percentage that could rise up to 15% by the age of 20[2]. The association of SUDEP with dysfunction in the voltage-gated sodium channel Nav1.1, encoded by the SCN1A gene, is emphasized by two studies that describe subjects carrying SCN1A variants who experienced sudden unexpected death in infancy and childhood despite having no history of seizures[3,4]. While the pathophysiology of SUDEP remains incompletely understood, insights from human studies and animal models propose alterations in cardiac, respiratory, and brainstem functions as plausible mechanistic contributors[5].
The expression of the SCN1A gene, encoding the α-subunit of the voltage-gated sodium channel Nav1.1, both in the brain and in the heart, raises the intriguing hypothesis that genetic mutations affecting sodium channel function may predispose individuals to both DS and arrhythmic disorders[6]. Coherently, DS patients exhibit depressed heart rate variability (HRV) and increased P- and QT-interval dispersion[7,8]. Moreover, a mouse model carrying the human mutation SCN1A-R1407X displayed premature death, prolonged QT intervals, and cardiac arrhythmias, which often precede spontaneous seizures, indicating potential neurocardiac or sole cardiac mechanisms contributing to sudden death[9].
Generalizing these data in the broader context of different sodium channel (SCN) genes, an interesting study investigated HRV in individuals with drug-resistant epilepsy with or without variants in SCN genes[10]. The authors found that in the whole population, SUDEP patients displayed severe autonomic dysregulation, characterized by lower awake HRV and abnormal sleep-to-awake HRV ratios. Interestingly, autonomic dysfunction was worse in subjects who harbored variants in SCN genes compared to patients with variants in other types of genes. Moreover, there is evidence for other specific so-called “cardiocerebral channelopathies”, such as SCN5A-related disorders, which are linked to epilepsy and long QT syndrome[11].
On the other hand, conflicting findings emerged from a multicenter study that recorded peri-ictal ECG of 547 seizures in DS patients, revealing no major arrhythmias but only a prevalent peri-ictal QTc-lengthening[2].
Therefore, considering the inconclusive data resulting from cardiac electrophysiology studies, recent research is shifting focus to respiratory function. Interestingly, a study on hypoxia and hypercapnia induced-QTc prolongation in healthy individuals, coupled with a study correlating peri-ictal QTc changes with SpO2, underscores the intricate relationship between respiratory and cardiac dynamics[12,13]. Accordingly, literature data demonstrate that, generally, in patients with drug-resistant epilepsy, there is an altered cardiac reactivity in response to sleep apnea[14]. More specifically, respiratory anomalies can be found in various developmental and epileptic encephalopathies (DEEs), not always related to seizures. For instance, respiratory issues are well-documented in patients with Rett syndrome and CDKL5-related DEE[15-17]. These patients exhibit episodes of hyperventilation followed by central apneas while awake, along with a reduced REM sleep duration, suggesting a failure of brainstem nuclei. Breathing problems are also present in KCNQ2-related DEE, likely due to impairment in Phox2b-expressing neurons in respiratory nuclei[18]. Finally, dysfunction in autonomic respiratory control has been observed in patients with West syndrome, who demonstrate less variable respiratory rates and a higher risk of apneas[19].
A recent elegant study tried to further elucidate this point in Dravet syndrome, combining clinical data from patients with experimental data from animal models[20]. The authors first showed that 7 patients with DS commonly have peri-ictal respiratory dysfunction, then reported a well-documented case of one patient who had severe and prolonged postictal hypoventilation during video EEG monitoring and died later of SUDEP. Subsequently, mice models of DS were monitored after spontaneous and heat-induced seizures, demonstrating that death was due to central apnea followed by progressive secondary bradycardia. The authors used pharmacological interventions to infer a possible pathogenic mechanism: anticholinergic drugs did not prevent bradycardia or death when given at doses selective for peripheral parasympathetic blockade, whereas apnea, bradycardia, and death were prevented by the same drugs given at doses high enough to cross the blood-brain barrier and to reach the autonomic regulatory nuclei located in the brainstem. Moreover, death was also prevented when an anticholinergic drug was given via intracerebroventricular infusion at a very low dose. These data suggest that SUDEP in patients with DS can result from primary central apnea.
To delve deeper into respiratory abnormalities in DS, we hereby present in detail the history of a girl with DS experiencing severe respiratory events not related to Sudden Infant Death Syndrome (SIDS), SUDEP, or fatal status epilepticus. Written informed consent was obtained from the patient’s parents. Moreover, we conducted a narrative review of reported cases with similar respiratory findings. Our main objective is to emphasize that some individuals with Dravet syndrome may show a significant predisposition to develop episodes of severe acute respiratory failure from even minor triggers, in the absence of pre-existing or subsequent lung damage.
CASE REPORT
The proband is a 4-year-old girl of Indian descent. At the age of 6 months, focal motor and non-motor seizures started, then followed by prolonged hemiclonic seizures, lasting 15-30 min, triggered by hot baths, fever, and vaccines. Dravet syndrome was suspected and a next-generation sequencing-based gene panel focusing on genes related to early-onset epileptic encephalopathies was performed. Levetiracetam was started while waiting for metabolic assessment and genetic results. After a few weeks, a de novo missense SCN1A gene mutation (c.4756G > A, p.Gly1586Arg) was found. Valproate and then clobazam were started and levetiracetam was slowly tapered. At the age of 10 months, the girl reached good seizure control, maintained for almost a year with only subtle non-motor seizures during febrile illnesses. At 18 months of age, the proband showed only mild language and social skills impairment, being otherwise healthy.
At 2 years of age, seizures relapsed, presenting with several episodes of prolonged, sometimes drug-resistant, generalized or focal motor (alternating) hemiclonic seizures, consistently triggered by fever or emotional stress. Seizures recurred roughly every 1-2 months. Among these, she presented three refractory or super-refractory status epilepticus (SE). The first one (aged 2 years 3 months) occurred while the girl was in India. She presented subsequent drug-resistant seizures during an upper respiratory infection, which required multiple anti-seizure medications (phenobarbital, topiramate) and admission to the pediatric intensive care unit (PICU) for monitoring. The second and third episodes (at 3 years 3 months and 3 years 6 months of age, respectively) were both characterized by febrile super-refractory status epilepticus, requiring anesthetic agents (propofol, ketamine, midazolam) for seizure control. At the age of 3 years and 7 months, stiripentol was added to prevent further episodes of febrile status epilepticus. At the last follow-up (4 years of age), the child was stably seizure-free since the introduction of stiripentol. Despite a phenotype consistent with DS, the clinical history of our patient is very peculiar. In fact, during the third year of age, she had recurrent episodes of hypoxemic respiratory failure (six in total) [Table 1]. All episodes occurred during fever and their remarkable severity often appeared disproportionate to the initial trigger. Common upper respiratory viruses were identified in 3/6 episodes [Table 1]. One episode occurred in India; pulmonary hypertension was diagnosed and acutely treated with sildenafil. Three out of six episodes required emergent intubation and mechanical ventilation; in one case, mechanical ventilation and inhaled nitric oxide were not sufficient due to severe refractory hypoxia, requiring support with venovenous extracorporeal membrane oxygenation (v-v ECMO) for one week [Table 1]. The relation between respiratory failure and epileptic seizures appeared to be inconsistent, as only three out of six episodes occurred concomitantly to seizures, while the remaining did not [Table 1].
Clinical characteristics of acute episodes of respiratory failure
Age | Fever | Trigger | Concomitant seizures | Maximum respiratory support | FiO2* | Duration of respiratory support (days) |
2 y 3 m | Yes | Not found | Subsequent seizures | NIV | n.a. | n.a. |
2 y 8 m | Yes | MIS-C secondary to COVID-19 | No | IMV iNO v-v ECMO | 100% | ECMO-7 IMV-14 TOT: 24 |
3 y | Yes | RSV | No | HNFC | 100% | HNFC-13 |
3 y 2 m | Yes | Metapneumovirus + bocavirus | No | HFNC | 28% | HNFC-13 |
3 y 3 m | Yes | Rhinovirus + enterovirus | Status epilepticus | IMV | 80% | IMV 12 TOT 23 |
3 y 6 m | Yes | Not found | Status epilepticus | IMV | 60% | IMV 9 TOT 13 |
Interestingly, after each acute episode, the girl fully recovered and her respiratory function returned to normal. Chest CT, performed during a period of well-being and clinical stability, showed no signs of chronic or primary pulmonary affection; respiratory monitoring, conducted during febrile illnesses but far from both severe acute respiratory episodes and seizures, showed rare, brief apneas without corresponding EEG correlates [Figure 1]. An extensive diagnostic work-up ruled out relevant comorbidities (cardiomyopathies, immunodeficiencies, metabolic diseases). Finally, whole exome sequencing was performed, with a particular focus on genes related to immune deficits and pulmonary hypertension. No relevant mutations other than SCN1A were found.
Figure 1. Respiratory polysomnography (age 3 years and 2 months) reveals brief apneas and shallow breathing with a concurrent reduction in oxygen saturation. Respiratory polysomnography was recorded in wakefulness simultaneously with EEG during a febrile infection (without concomitant seizures) but in a phase of relative well-being. It demonstrates an approximately 6-second apnea (leftmost panel) corresponding to an oxygen saturation of 95%. This brief apnea is followed by a phase of shallow breathing, during which saturation decreases to 91%, as highlighted by the two panels on the right. These two alterations in the respiratory pattern do not show an ictal EEG correlation. EEG data: High-pass filter 70 Hz, low-pass filter 1.6 Hz, notch filter 50 Hz, amplitude 100 uV/cm. ECG: Electrocardiogram; PNG: pneumogram; DelD: polygraph on right deltoid muscle; DelS: polygraph on left deltoid muscle; PULS: peripheral pulse; BEAT: heart frequency; SpO2: oxygen saturation.
Considering the severity of the respiratory episodes and the recurrent PICU admissions, a multidisciplinary team consisting of pediatric neurologists, pediatric intensivists, and pulmonologists recommended a tracheostomy as a preventive strategy to facilitate respiratory support in case of relapses. After parental consent, a tracheostomy was performed at the age of three years and seven months and night-time ventilation was initiated. Since then, the child did not present severe respiratory events despite the onset of another febrile episode (etiological agent not identified).
MATERIALS AND METHODS
We conducted a literature review via PubMed and SCOPUS (up to August 2024), using the following search string: (Dravet OR “NAV1.1 Voltage-Gated Sodium Channel”[Mesh] OR SCN1A OR Severe Myoclonic Epilepsy of Infancy OR SMEI) AND (“Pneumonia”[Mesh] OR “Respiratory Distress Syndrome”[Mesh] OR Respiratory OR Pulmonary OR ECMO OR “Extracorporeal Membrane Oxygenation”[Mesh] OR “Tracheostomy”[Mesh]). A manual reference check of the retrieved articles was also performed. Studies published in languages other than English were excluded.
We included in this review all data with available clinical details about individuals with DS (both children and adults) who suffered from severe respiratory events that were not related to SIDS, SUDEP, acute encephalopathy, fatal status epilepticus or due to drugs’ side effects.
RESULTS
In our comprehensive narrative literature review, we identified three case series and four case reports detailing instances of severe respiratory events in children with SCN1A-DS, within an age range of 1.2-15 years, encompassing a total of 21 cases (summarized in Table 2, along with our case report). To the best of our knowledge, no adult subjects have been reported so far. Most respiratory events occurred in the context of respiratory infections of variable severity, ranging from dyspnea with respiratory distress requiring O2 supplementation to severe and refractory ARDS necessitating PICU admission and maximal life support. In milder cases or where specialized home care was available, children were successfully treated at home. However, due to the severity of respiratory impairment, hospitalization was needed in 19/21 and PICU admission in six cases. In 15/21 cases (71.4%), a high temperature was reported, while information was incomplete in five cases. An infectious agent was identified for 8/21, the most common being Influenza A virus (5) and Adenovirus (2). The presence of concomitant seizures or status epilepticus was specified only in 8 cases, while data were incomplete in most of the remaining. The outcome was fatal for 3 children, aged 5-11 years, who died of refractory respiratory insufficiency and multiple complications (including pleural diffusion, hypotension, and multi-organ failure), despite high-intensity care. In instances where an encephalopathic state was observed, children survived with severe neurological sequelae. One patient, described by Howell et al. (2023), required a tracheostomy placement after experiencing two subsequent influenza infections associated with encephalopathy[21]. Only in one case was the respiratory event described as a peri-ictal respiratory dysfunction rather than an infection[20].
Clinical characteristics of Dravet patients with severe respiratory events
Author, date | Gender, age (y) | Respiratory event | Infectious agent | Fever | Concomitant seizures and/or SE | Care setting | Maximal respiratory support | Complications | Death |
Nosaka et al., 2015[22] | F, (1.2) | Severe ARDS | n.d. | Yes | Yes | PICU | ECMO | HFOV-related pneumothorax, fluid overload | No |
Tziouvas et al., 2018[26] | F, (9) | Respiratory insufficiency | n.a. | Yes | Yes | Hospital | High O2 concentration | Sepsis, MOF | Yes |
Tziouvas et al., 2018[26] | M, (6) | Pneumonia with respiratory insufficiency | n.a. (bacterial) | Yes | Yes | Hospital | High O2 concentration | Pleural empyema | No |
Tziouvas et al., 2018[26] | M, (3) | Pneumonia with respiratory insufficiency | Adenovirus | Yes | Yes | Hospital | High O2 concentration | Lung consolidation | No |
Tziouvas et al., 2018[26] | F, (15) | Pneumonia with respiratory insufficiency | Influenza A | Yes | Yes | Hospital | High O2 concentration | No | No |
Tziouvas et al., 2018[26] | n.a., (6) | Respiratory insufficiency | n.d. | Yes | Yes | Hospital | High O2 concentration | No | No |
Tziouvas et al., 2018[26] | n.a., (6) | Respiratory insufficiency | n.d. | Yes | Yes | Hospital | High O2 concentration | No | No |
Yeşilbaş et al., 2018[23] | M, (5) | Bronchopneumonia, ARDS | Adenovirus | Yes | No | PICU | ECMO | Pleural effusion, MOF, pneumomediastinum | Yes |
Kim et al., 2018[20] | F, (9) | Apnea, hypoventilation | no | No | Yes | Hospital | O2 supplementation | n.a. | No* |
Balestrini et al., 2021[25] | n.a. (< 5) | Respiratory insufficiency | n.a. | n.a. | n.a. | Hospital | Resuscitation and ventilation | Increased frequency and duration of seizures | No |
Balestrini et al., 2021[25] | n.a., (< 5) | Dyspnea | n.a. | n.a. | n.a. | Hospital | O2 supplementation | Increased frequency of seizures | No |
Balestrini et al., 2021[25] | n.a., ( < 5) | n.a. | n.a. | Yes | n.a. | Hospital | Ventilation | Increased frequency and duration of seizures | No |
Balestrini et al., 2021[25] | n.a., (5-11) | n.a. | Probable COVID-19 | Yes | n.a. | Home | O2 supplementation | Increased frequency of seizures | No |
Balestrini et al., 2021[25] | n.a., (5-11) | Dyspnea | n.a. | Yes | n.a. | Home | O2 supplementation | no | No |
Balestrini et al., 2021[25] | n.a., (5-11) | n.a. | n.a. | Yes | n.a. | Home | O2 supplementation (already on BiPAP) | no | No |
Balestrini et al., 2021[25] | n.a., (5-11) | Dyspnea | n.a. | Yes | n.a. | Hospital | O2 supplementation | Increased frequency and duration of seizures | No |
Howell et al., 2023[21] | F, (11) | Pneumonia | Influenza A | Yes | n.a. | PICU | n.a. | Hypotension | Yes |
Howell et al., 2023[21] | M, (4) | Pneumonia | Influenza A | n.a. | Yes | PICU | n.a. | Encephalopathy | No |
Howell et al., 2023[21] | M, (8) | ARDS | Influenza A | n.a. | n.a. | PICU | n.a. (tracheostomy) | Encephalopathy, surgical emphysema | No |
Howell et al., 2023[21] | F, (8) | ARDS | Influenza A | n.a. | Yes | PICU | n.a. | Encephalopathy, hypotension, AKI | No |
Tran et al., 2024[24] | F, (2) | Pneumonia with respiratory distress | RSV | Yes | No | Hospital | O2 supplementation (nasal cannula) | Dehydration, malnutrition (PEG placement), metabolic acidosis | No |
Favaro, Ancora | F, (4) | Recurrent ARDS | RSV, rhinovirus, enterovirus, metapneumovirus, bocavirus, COVID-19 | Yes | Inconstantly associated | PICU | ECMO | Pulmonary hypertension | No |
Specifically, Nosaka et al. (2015) provided detailed insights into a 15-month-old child admitted with febrile status epilepticus[22]. Seizures rapidly subsided once the temperature was controlled. However, the patient underwent intubation for airway protection and received antibiotics for suspected aspiration pneumonia, subsequently developing acute respiratory distress syndrome (ARDS). Despite the maximization of ventilatory parameters, synchronized intermittent mandatory ventilation proved insufficient, requiring a transition to high-frequency oscillatory ventilation and, subsequently, venoarterial ECMO. A dose of surfactant was administered. After 15 days, the girl was successfully weaned off ECMO and later discharged without additional neurological deterioration. Notably, the article did not speculate further on potential etiologies and whether echocardiography was performed to rule out pulmonary hypertension.
The specific etiological agent was identified in the following case reports. Yeşilbaş et al. (2018) discussed a 5-year-old child with DS admitted for Adenovirus bronchopneumonia[23]. Despite appropriate mechanical ventilation, the child rapidly developed ARDS, showing insufficient oxygenation. Unfortunately, regardless of the initiation of ECMO therapy, the child died shortly afterward.
As previously mentioned, Howell et al. (2023) described the complications associated with Influenza infections in individuals with DS[21]. In this work, among a population of 82 subjects followed at Australian specialized Dravet clinics, 21 had confirmed Influenza infections, all of whom required hospital admission mostly due to seizure exacerbation. Furthermore, in four cases, severe respiratory symptoms occurred, including ARDS and pneumonia, proving fatal in one patient.
Finally, Tran et al. (2024) reported the clinical course of an infant with RSV-related pneumonia and respiratory distress who required hospitalization and oxygen supplementation, along with percutaneous endoscopic gastrostomy (PEG) implantation for malnutrition and severe dehydration[24].
Moving on, Balestrini et al. (2021) conducted a cross-sectional survey to investigate the effects of COVID-19 on the DS population[25]. In this study, a total of 19 out of 116 individuals presented with respiratory symptoms during the COVID-19 pandemic. Although COVID-19 was suspected, none of the individuals underwent specific testing, except for one patient who tested positive for antibodies. Seven patients developed significant respiratory difficulties, requiring different levels of home and hospital care, ranging from the supplementation of additional oxygen to the use of mechanical ventilation. The outcome was globally favorable, although in most cases, an increase in frequency and/or duration of seizures was observed.
Regardless of specific infectious etiologies, Tziouvas et al.’s work (2018) outlined the predisposition of individuals with DS to develop respiratory insufficiency in the context of intercurrent infections, requiring intensive care and leading to increased morbidity[26]. Six children with DS, facing febrile illnesses with concomitant seizures, were briefly reported. These children required a high concentration of oxygen to maintain a normal saturation even after achieving seizure control. Three were diagnosed with pneumonia, while the remaining experienced prolonged (> 2 days) respiratory insufficiency without evidence of pulmonary infection. The outcome was fatal for a girl who developed sepsis and MOF.
Lastly, the paper presented by Kim et al. (2018) aimed to more accurately characterize abnormalities in peri-ictal breathing in DS[20]. They analyzed video recordings of generalized seizures of seven subjects with DS, comparing them with those of patients with structural epilepsy, disclosing that severe peri-ictal breathing abnormalities were significantly more frequent in DS, including disturbances in respiratory rhythm and output. They then detailed the case of a 9-year-old girl with DS. The child underwent a prolonged video-EEG recording, supplemented with respiratory impedance plethysmography and transcutaneous CO2 (tcCO2) recordings documenting dyspnea and brief periods of apnea. Fourty-five min after the third seizure, the patient developed labored breathing, oxygen desaturation, and hypercapnia. Three years after the recording, the patient was found prone, cyanotic, and pulseless in bed and could not be resuscitated.
DISCUSSION
The extensively studied case we have reported raises fundamental questions regarding respiratory alterations in DS patients, particularly when considering its peculiar aspects in conjunction with the limited literature on this topic.
First, the severe episodes of respiratory failure observed in our patient were not constantly associated with status epilepticus or refractory seizures. Instead, they consistently occurred in the context of intercurrent febrile infections, mostly of presumed viral origin, although not always with a clearly identified etiological agent. Moreover, the symptoms exhibited by the patient were disproportionately severe compared to the extent and nature of the infection. Chest CT scan and pulmonary function tests ruled out the presence of pre-existent or subsequent lung damage, excluding that a lung injury could be part of the specific phenotype of our subject. Furthermore, immunological tests did not reveal any immune deficiencies and exome sequencing ruled out genetically determined immunodeficiencies or pulmonary hypertension. These data point to autonomic dysregulation and an impaired homeostatic process. Consequently, we conducted a literature search to gather DS cases where severe respiratory abnormalities were associated with respiratory febrile infections. The data we have collected, however, are not entirely conclusive.
The 21 subjects with DS identified experienced respiratory events of varying severity, ranging from dyspnea with respiratory distress requiring O2 supplementation to severe and refractory ARDS necessitating PICU admission and maximal life support. Hospitalization was necessary in 90% of cases, and PICU admission in 29%. Despite receiving high-intensity care, three subjects had an unfavorable outcome and passed away.
On the other hand, surveys conducted in the UK and within European patient advocacy groups did not find an increased rate of severe respiratory events in DS patients during the COVID-19 pandemic[25,27]. Conversely, an Australian study conducted by Howell et al. (2023) showed that in a population of 24 individuals with DS who contracted influenza, 21% developed severe complications such as pneumonia and ARDS[21]. Furthermore, a Swedish population-based study on DS reported that three out of 53 individuals died of pulmonary infections, and two others died possibly due to SUDEP associated with a respiratory infection, although a comprehensive clinical history was not available for these patients[28]. Nonetheless, in previous large studies, mortality rates due to infections appeared comparatively smaller[2,29-32].
Then, considering that in our patient, three out of six episodes of respiratory failure occurred concomitantly with seizures or status epilepticus, we searched the literature for a possible association between respiratory infections, status epilepticus, and severe and prolonged respiratory abnormalities. A survey conducted in Europe through Dravet Italia Onlus and the EpiCARE network in 2020[33] collected data on seven individuals with DS who died following refractory SE. Fatal SE was consistently triggered by fever, either due to respiratory infection or, in one case, hexavalent vaccination. The death trajectory varied: four patients died during or shortly after the onset of SE, while three experienced a progression into a coma-like state, culminating in death several days later. Early neuroradiological findings in three patients suggested cerebral edema as a potential ultimate cause of death, with no specific respiratory data reported. In this perspective, Okumura et al. (2012) clarified for the first time the features of acute encephalopathy in children with DS, stating that despite hypoxic-ischemic damage possibly explaining widespread cerebral edema, serious hypoxia and/or systemic circulatory failure were not observed in any but one of their 15 patients during the first few days of febrile illness[34]. Another series of five children with DS who died following febrile status epilepticus was reported by Myers et al. (2017)[35]. These five subjects experienced convulsive status epilepticus associated with fever and a suspected or confirmed viral infection and required intubation and ventilation due to multi-organ dysfunction. All demonstrated dramatic cerebral and cerebellar edema, far more severe than imaging changes typically seen after convulsive status epilepticus. The authors suggested that hypoxic-ischemic insult might have contributed to this diffuse edema; however, this would not be the only cause, as the edema was not confined to the typical watershed areas. On the other hand, De Liso et al. (2020) suggested a possible pathophysiological sequence of events involving hyperthermia-induced epileptic seizures, leading to postictal respiratory failure and cardiac arrest, subsequently resulting in cerebral edema[33]. Interestingly, our patient did not progress into a coma-like state and we did not observe either a disorganization of brain electrical activity or an edema on the MRI. This observation raises the possibility that severe respiratory failure and acute encephalopathy may not always be associated.
It is also important not to focus exclusively on apneas and hypopneas. For example, a study by Dhamija et al. (2014) that focused on nocturnal polysomnographic data of six children with Dravet Syndrome did not show any consistent abnormalities in the obstructive or central apnea index[36]. In this context, another important aspect of discussion raised by our case report concerns the severity and persistence of hypoxemia. In fact, in our patient, hypoxia tended to persist for a long time, even during mechanical ventilation, despite the normalization of pCO2. This noteworthy aspect appears to be consistent with other cases we have reported and suggests a long-term respiratory impairment following both SE and respiratory infection. It is plausible, therefore, that two overlapping mechanisms are at play: the first is related to a reduction in ventilation, and the second is more strongly associated with an alteration in gas exchange. Supportive of the first mechanism are findings on interictal EEG, revealing brief apneas without corresponding electrical correlates and X-ray evidence of under-recruited lungs. Supportive of the second mechanism is the identification of pulmonary hypertension during the initial episode in India. However, pulmonary hypertension was not confirmed by echocardiography during subsequent episodes and exome sequencing ruled out the presence of mutations in genes associated with congenital pulmonary hypertension.
As evident from the aforementioned animal models, anticholinergic drugs prevent apnea, bradycardia, and death only when administered at doses high enough to cross the blood-brain barrier, suggesting that hypoventilation is driven by a central nervous system mechanism, like in primary central apnea[20]. Moreover, it is worth noting that approximately 60%-80% of patients with SE may suffer from neurogenic pulmonary edema, and the incidence is 100% in patients who die from seizures[37]. Similar to our case, in these patients, the results of the ultrasonic cardiogram, transesophageal Doppler, and central venous pressure are typically within normal limits and in survivors, recovery is usually rapid with appropriate management. Several studies have shown that neurogenic pulmonary edema is closely related to specific central nervous system centers critical for the regulation of the autonomic nervous system located within the insular cortex, hypothalamus, and medulla oblongata[37-39]. Specifically, the trigger zones in the brainstem are closely related to C1 neurons thought to regulate the autonomic nervous system via projections to sympathetic and parasympathetic preganglionic neurons[38,40,41]. In support of the hypothesis of sympatho-vagal imbalance, interictal data in individuals with DS showed decreased heart rate variability[8,10], an index that, when low, indicates excessive sympathetic activity and/or low parasympathetic activity. Another interesting finding is that decreased heart rate variability due to abnormal vagal activity is associated with increased levels of inflammatory markers[42]. During respiratory infection, the local release of pro-inflammatory mediators can stimulate afferent vagal nerve fibers. At the level of the brainstem regulatory centers, the inflammatory reflex mediated by the vagal nerve and aimed at toning down inflammation is not evoked, triggering a vicious cycle leading to further production of inflammatory cytokines. These, in turn, can lead to endothelial damage and increased capillary permeability in the lungs, generating edema. Conversely, an overactivation of the sympathetic system can occur, and due to pronounced sympathetic vasoconstriction, blood is redistributed from systemic vascular beds to the pulmonary circulation. The increase in central blood volume and pulmonary vascular pressure results in a fluid shift into the interstitial space.
The hypothesis arising from the aforementioned mechanisms is that individuals with DS may have a primary alteration affecting the autonomic regulatory nuclei, leading to an imbalance between sympathetic and parasympathetic tone, as evidenced by data on heart rate variability. In the case of infection, especially respiratory infections, this imbalance may worsen further. This could result in both apnea with potentially fatal arrhythmias and neurogenic pulmonary edema, which could justify prolonged and challenging-to-treat hypoxemia.
CONCLUSIONS
In conclusion, the presented case study and comprehensive literature review provide insights into critical aspects of respiratory abnormalities in individuals with DS, contributing to our understanding of potential life-threatening complications in these patients. The observed severe respiratory events in DS cases, particularly during febrile intercurrent infections, raise intriguing questions about the interplay between autonomic regulatory nuclei, inflammatory responses, and pulmonary dynamics. The discrepancy between the severity of respiratory symptoms and the nature of the infection, as well as the persistence of hypoxemia during mechanical ventilation, highlights the need for further exploration into the underlying pathophysiology. The literature review, encompassing case reports and series, underscores the importance of tailored clinical management and vigilant monitoring during febrile illnesses, considering the potential for both apnea-related arrhythmias and prolonged, challenging-to-treat hypoxemia. The proposed hypothesis of autonomic dysregulation offers a potential framework for understanding the complex relationship between neurological, cardiac, and respiratory functions. Moving forward, continued research, including longitudinal studies and advanced physiological monitoring, is crucial to refine our understanding of respiratory abnormalities in DS. Moreover, improved insights into the pathophysiological mechanisms will inform targeted interventions, enhance clinical care, and potentially reduce the risk of life-threatening events in individuals with DS.
DECLARATIONS
Acknowledgments
The authors thank the patient and her family for granting permission to use the clinical data. Thanks to Gioele Geremia Paolin for his kind contribution.
Authors’ contributions
Clinical management of the patient: Favaro J, Ancora C, Brunello F, Paolin C, Cavaliere E, Carraro S, Poskurica I, Pegoraro V, Cogo P, Bonardi CM, Pelizza MF, Pin JN, Sartori S, Toldo I
Conceptualization: Favaro J, Ancora C, Brunello F, Paolin C, Cavaliere E, Carraro S, Sartori S, Toldo I
Resources and data curation: Brunello F, Paolin C, Cavaliere E, Poskurica I, Pegoraro V
Writing - original draft: Favaro J, Ancora C, Brunello F
Writing - review & editing: Favaro J, Toldo I
Literature review: Ancora C, Paolin C
Supervision: Carraro S, Cogo P, Sartori S, Toldo I
Availability of data and materials
Not applicable.
Financial support and sponsorship
None.
Conflicts of interest
All authors declared that there are no conflicts of interest.
Ethical approval and consent to participate
Not applicable.
Consent for publication
Written informed consent for publication of the clinical details, images, and any other relevant information was obtained from the patient’s parents. The family was fully informed about the nature of the study and the potential implications of the publication, and agreed to the use of this information in this publication.
Copyright
© The Author(s) 2024.
REFERENCES
1. Scheffer IE, Nabbout R. SCN1A-related phenotypes: epilepsy and beyond. Epilepsia 2019;60 Suppl 3:S17-24.
2. Shmuely S, Sisodiya SM, Gunning WB, Sander JW, Thijs RD. Mortality in dravet syndrome: a review. Epilepsy Behav 2016;64:69-74.
3. Brownstein CA, Goldstein RD, Thompson CH, et al. SCN1A variants associated with sudden infant death syndrome. Epilepsia 2018;59:e56-62.
4. Halvorsen M, Petrovski S, Shellhaas R, et al. Mosaic mutations in early-onset genetic diseases. Genet Med 2016;18:746-9.
5. Bagnall RD, Crompton DE, Semsarian C. Genetic basis of sudden unexpected death in epilepsy. Front Neurol 2017;8:348.
6. Daverio M, Ciccone O, Boniver C, De Palma L, Corrado D, Vecchi M. Supraventricular tachycardia during status epilepticus in dravet syndrome: a link between brain and heart? Pediatr Neurol 2016;56:69-71.
7. Delogu AB, Spinelli A, Battaglia D, et al. Electrical and autonomic cardiac function in patients with Dravet syndrome. Epilepsia 2011;52 Suppl 2:55-8.
8. Ergul Y, Ekici B, Tatli B, Nisli K, Ozmen M. QT and P wave dispersion and heart rate variability in patients with Dravet syndrome. Acta Neurol Belg 2013;113:161-6.
9. Auerbach DS, Jones J, Clawson BC, et al. Altered cardiac electrophysiology and SUDEP in a model of Dravet syndrome. PLoS One 2013;8:e77843.
10. Myers KA, Bello-Espinosa LE, Symonds JD, et al. Heart rate variability in epilepsy: a potential biomarker of sudden unexpected death in epilepsy risk. Epilepsia 2018;59:1372-80.
11. Remme CA. SCN5A channelopathy: arrhythmia, cardiomyopathy, epilepsy and beyond. Philos Trans R Soc Lond B Biol Sci 2023;378:20220164.
12. Roche F, Reynaud C, Pichot V, et al. Effect of acute hypoxia on QT rate dependence and corrected QT interval in healthy subjects. Am J Cardiol 2003;91:916-9.
13. Goldenholz DM, Kuhn A, Austermuehle A, et al. Long-term monitoring of cardiorespiratory patterns in drug-resistant epilepsy. Epilepsia 2017;58:77-84.
14. Ricordeau F, Chouchou F, Pichot V, et al. Impaired post-sleep apnea autonomic arousals in patients with drug-resistant epilepsy. Clin Neurophysiol 2024;160:1-11.
15. Cherchi C, Chiappini E, Amaddeo A, et al. panel for the Problems in Patients with Rett Syndrome. Management of respiratory issues in patients with Rett syndrome: Italian experts’ consensus using a Delphi approach. Pediatr Pulmonol 2024;59:1970-8.
16. Hagebeuk EEO, Smits A, de Weerd A. Long time polysomnographic sleep and breathing evaluations in children with CDKL5 deficiency disorder. Sleep Med 2023;103:173-9.
17. Hagebeuk EE, van den Bossche RA, de Weerd AW. Respiratory and sleep disorders in female children with atypical Rett syndrome caused by mutations in the CDKL5 gene. Dev Med Child Neurol 2013;55:480-4.
18. Soto-Perez J, Cleary CM, Sobrinho CR, et al. Phox2b-expressing neurons contribute to breathing problems in Kcnq2 loss- and gain-of-function encephalopathy models. Nat Commun 2023;14:8059.
19. Jansen K, Varon C, Van Huffel S, Lagae L. Early respiratory dysfunction as a biomarker for epileptic encephalopathy. Acta Neurol Scand 2013;128:381-5.
20. Kim Y, Bravo E, Thirnbeck CK, et al. Severe peri-ictal respiratory dysfunction is common in Dravet syndrome. J Clin Invest 2018;128:1141-53.
21. Howell KB, Butcher S, Schneider AL, et al. Complications of influenza A or B virus infection in individuals with SCN1A-positive Dravet syndrome. Neurology 2023;100:E435-42.
22. Nosaka N, Ichiba S, Tsukahara K, et al. Acute respiratory distress syndrome in a child with severe epileptic disorder treated successfully by extracorporeal membrane oxygenation: a case report. BMC Pediatr 2015;15:29.
23. Yeşilbaş O, Şevketoğlu E, Kıhtır HS, et al. Fatal adenovirus pneumonia despite extracorporeal life support treatments. J Pediatr Infect 2018;12:73-7.
24. Tran NN, Liu J, Bullock T, Flowers D. Respiratory syncytial virus in a child with dravet syndrome: a case report. Cureus 2024;16:e59405.
25. Balestrini S, Wilson G, Eldred C, Evans H, Sisodiya SM. The impact of COVID-19 in Dravet syndrome: a UK survey. Acta Neurol Scand 2021;143:389-95.
26. Tziouvas K, Machaira M, Goula E, Vartzelis G, Papadatos J. Abstract P-516: respiratory insufficiency or infections as a common cause for morbidity in Dravet syndrome. Pediatr Crit Care Me 2018;19:208.
27. Brambilla I, Aibar JÁ, Hallet AS, et al. Impact of the COVID-19 lockdown on patients and families with Dravet syndrome. Epilepsia Open 2021;6:216-24.
28. Bjurulf B, Reilly C, Sigurdsson GV, Thunström S, Kolbjer S, Hallböök T. Dravet syndrome in children-a population-based study. Epilepsy Res 2022;182:106922.
29. Cooper MS, Mcintosh A, Crompton DE, et al. Mortality in Dravet syndrome. Epilepsy Res 2016;128:43-7.
30. Sakauchi M, Oguni H, Kato I, et al. Retrospective multiinstitutional study of the prevalence of early death in Dravet syndrome. Epilepsia 2011;52:1144-9.
31. Skluzacek JV, Watts KP, Parsy O, Wical B, Camfield P. Dravet syndrome and parent associations: the IDEA league experience with comorbid conditions, mortality, management, adaptation, and grief. Epilepsia 2011;52 Suppl 2:95-101.
32. Akiyama M, Kobayashi K, Yoshinaga H, Ohtsuka Y. A long-term follow-up study of Dravet syndrome up to adulthood. Epilepsia 2010;51:1043-52.
33. De Liso P, Pironi V, Mastrangelo M, et al. Fatal Status Epilepticus in Dravet syndrome. Brain Sci 2020;10:889.
34. Okumura A, Uematsu M, Imataka G, et al. Acute encephalopathy in children with Dravet syndrome. Epilepsia 2012;53:79-86.
35. Myers KA, McMahon JM, Mandelstam SA, et al. Fatal cerebral edema with status epilepticus in children with Dravet syndrome: report of 5 cases. Pediatrics 2017;139:e20161933.
36. Dhamija R, Erickson MK, St Louis EK, Wirrell E, Kotagal S. Sleep abnormalities in children with Dravet syndrome. Pediatr Neurol 2014;50:474-8.
37. Yang A, Liu B, Inoue T. Role of autonomic system imbalance in neurogenic pulmonary oedema. Eur J Neurosci 2022;55:1645-57.
38. Baumann A, Audibert G, McDonnell J, Mertes PM. Neurogenic pulmonary edema. Acta Anaesthesiol Scand 2007;51:447-55.
39. Bonaz B, Sinniger V, Pellissier S. Vagal tone: effects on sensitivity, motility, and inflammation. Neurogastroenterol Motil 2016;28:455-62.
40. Darragh TM, Simon RP. Nucleus tractus solitarius lesions elevate pulmonary arterial pressure and lymph flow. Ann Neurol 1985;17:565-9.
41. Inobe JJ, Mori T, Ueyama H, Kumamoto T, Tsuda T. Neurogenic pulmonary edema induced by primary medullary hemorrhage: a case report. J Neurol Sci 2000;172:73-6.
Cite This Article

How to Cite
Favaro, J.; Ancora, C.; Brunello, F.; Paolin, C.; Cavaliere, E.; Carraro, S.; Poskurica, I.; Pegoraro, V.; Cogo, P.; Bonardi, C. M.; Pelizza, M. F.; Pin, J. N.; Sartori, S.; Toldo, I. Respiratory abnormalities in Dravet syndrome: insights from a case report and literature review. Rare. Dis. Orphan. Drugs. J. 2024, 3, 30. http://dx.doi.org/10.20517/rdodj.2023.64
Download Citation
Export Citation File:
Type of Import
Tips on Downloading Citation
Citation Manager File Format
Type of Import
Direct Import: When the Direct Import option is selected (the default state), a dialogue box will give you the option to Save or Open the downloaded citation data. Choosing Open will either launch your citation manager or give you a choice of applications with which to use the metadata. The Save option saves the file locally for later use.
Indirect Import: When the Indirect Import option is selected, the metadata is displayed and may be copied and pasted as needed.
About This Article
Copyright
Data & Comments
Data
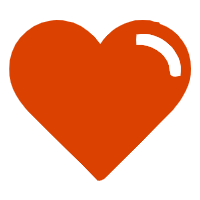
Comments
Comments must be written in English. Spam, offensive content, impersonation, and private information will not be permitted. If any comment is reported and identified as inappropriate content by OAE staff, the comment will be removed without notice. If you have any queries or need any help, please contact us at support@oaepublish.com.