Compendium of Bifidobacterium-based probiotics: characteristics and therapeutic impact on human diseases
Abstract
The human microbiota, a complex community of microorganisms residing in and on the human body, plays a crucial role in maintaining health and preventing disease. Bifidobacterium species have shown remarkable therapeutic potential across a range of health conditions, thus being considered optimal probiotic bacteria. This review provides insights into the concept of probiotics and explores the impact of bifidobacteria on human health, focusing on the gastrointestinal, respiratory, skeletal, muscular, and nervous systems. It also integrates information on the available genetic bases underlying the beneficial effects of each bifidobacterial probiotic species on different aspects of human physiology. Notably, Bifidobacterium-based probiotics have proven effective in managing gastrointestinal conditions such as constipation, antibiotic-associated diarrhea, irritable bowel syndrome (IBS), inflammatory bowel disease (IBD), and Helicobacter pylori infections. These benefits are achieved by modulating the intestinal microbiota, boosting immune responses, and strengthening the gut barrier. Moreover, Bifidobacterium species have been reported to reduce respiratory infections and asthma severity. Additionally, these probiotic bacteria offer benefits for skeletal and muscular health, as evidenced by Bifidobacterium adolescentis and Bifidobacterium breve, which have shown anti-inflammatory effects and symptom relief in arthritis models, suggesting potential in treating conditions like rheumatoid arthritis. Furthermore, probiotic therapies based on bifidobacterial species have shown promising effects in alleviating anxiety and depression, reducing stress, and enhancing cognitive function. Overall, this review integrates the extensive scientific literature now available that supports the health-promoting applications of probiotic Bifidobacterium species and underscores the need for further research to confirm their clinical efficacy across different body systems.
Keywords
INTRODUCTION
Multicellular organisms, including humans, inhabit in association with a vast number of microorganisms, including bacteria, archaea, viruses, and unicellular eukaryotes. This complex microbial community, collectively known as the microbiota, is involved in a symbiotic relationship with its host. Within the human body, the microbiota exerts critical roles across a variety of physiological processes, including nutrient metabolism and immune system modulation[1,2]. These interactions are supposed to be vital for maintaining health and preventing disease, demonstrating the key role exploited by the microbiota in host well-being[3-5].
The human body harbors distinctive microbial communities across its various anatomical sites, which are adapted to the unique environmental conditions of the specific body site[6]. Distinct microbiotas are found, for example, on the skin or internal body sites such as the mouth, the respiratory tract, the gastrointestinal tract, and the female reproductive tract, each fulfilling specialized functions[7,8].
Due to its complexity and diversity, the intestinal microbiota represents a target of marked scientific interest among these diverse microbial habitats. This dense microbial community is considered to play a critical role in the host’s well-being, performing several beneficial functions. The intestinal microbiota is supposed to be involved in crucial metabolic processes, including carbohydrate digestion and vitamin synthesis[1]. Furthermore, it is supposed to play an important role in the development of immune responses and in safeguarding the host against colonization by pathogenic bacteria[9,10].
The composition of the intestinal microbiota is influenced by a series of endogenous features closely related to the host and environmental factors, such as age, sex, diet, antibiotic treatment, and lifestyle[11-14].
Maintaining the balance of the intestinal microbiota is crucial for health, as this symbiotic relationship can be disrupted by events that change the composition of the microbiota, leading to dysbiosis. Dysbiosis is characterized by an imbalance in the gut's microbial ecosystem[15]. Numerous studies have highlighted a correlation between intestinal dysbiosis and several human diseases/disorders, such as inflammatory bowel disease (IBD), irritable bowel syndrome (IBS), type 2 diabetes (T2D), celiac disease, and obesity[10,16,17]. In this context, preserving and restoring the intestinal microbiota balance is crucial for maintaining overall human health[18].
Currently, this modulation can occur through various methods and interventions, each capable of influencing the composition and activity of the microbes within our bodies [Figure 1A]. Among these, diet plays an important role in modulating the intestinal microbiota. In fact, changes in dietary habits can profoundly impact the types of microbes residing in the gut. Thus, certain foods can promote the growth of beneficial bacteria, while others may promote the proliferation of harmful microorganisms[19]. In addition, probiotics and prebiotics, i.e., compounds that stimulate the growth of beneficial bacteria, are considered to play a crucial role in shaping the microbiota composition and promoting gut health[20,21].
Figure 1. Illustration of potential modulators and general mechanisms influencing gut microbiota composition and host health. Panel (A) illustrates the potential modulators of the human microbiota. Specifically, modulators that promote eubiosis are highlighted in green, while modulators that contribute to dysbiosis are highlighted in red; Panel (B) shows several mechanisms through which bifidobacteria exert beneficial effects on the host. Specifically, bifidobacteria strengthen the intestinal barrier by increasing mucus production and preventing the colonization of opportunistic pathogens, thereby protecting against infections (a). Additionally, bifidobacteria interact with the host’s immune system, stimulating the production of anti-inflammatory cytokines that regulate immune responses and reduce inflammation (b). Bifidobacteria also produce neuroactive molecules, such as GABA, which may influence the nervous system, alleviating stress and supporting mental well-being (c). Furthermore, bifidobacteria generate metabolites like lactate and acetate, which can be utilized by other gut bacteria, such as SCFA-producing bacteria, to synthesize beneficial compounds like butyrate and propionate, contributing to gut health and metabolic regulation (d). GABA: Gamma-aminobutyric acid; SCFA: short-chain fatty acid.
Moreover, in recent years, an innovative approach known as fecal microbiota transplantation (FMT) has emerged as a promising intervention for restoring healthy microbiota in individuals with gut disorders or conditions related to microbial imbalance[22-28]. In detail, FMT involves introducing processed fecal material from a healthy donor to the recipient to reintroduce a diverse array of beneficial microbes, thereby promoting gut health and overall well-being. Numerous studies have highlighted the efficacy of this treatment and its therapeutic potential across various pathologies, with the main target being the treatment for recurrent Clostridioides difficile infection[29]. Nonetheless, concerns about disease transmission, recipient immune response, potential adverse effects, lack of standardization, absence of unified legislation, high costs, and invasiveness emphasize the importance of further refining FMT as a therapeutic approach, making it still largely experimental in most cases[30].
Among the various approaches modulating the intestinal microbiota, probiotic microorganisms are the most utilized due to their applicability and less invasive nature while still being effective. Probiotics aim to restore intestinal homeostasis by promoting the colonization of beneficial bacteria and restricting the expansion of pathogenic bacteria, thereby preventing dysbiosis conditions[31]. Lactobacilli and bifidobacteria are the most prevalent and widely used among all probiotic organisms[32,33]. Bifidobacteria are well-documented for their health benefits and have co-evolved with humans, playing a fundamental role in the early gut microbiota[34,35]. In fact, bifidobacteria are considered essential for “educating” the immune system stimulating and supporting gut physiology, such as mucin production[36-38]. Likewise, these microorganisms could inhabit the human gut due to the host’s production of prebiotic molecules like mucin, which supports their survival and colonization[37,39]. This relationship and the demonstrated transmission of these microorganisms from mother to child through vertical transmission mechanisms[40] underscore the co-evolution of bifidobacteria with their human host. The decrease in this bacterial population within both children’s and adult’s gut microbiota has been negatively correlated with numerous diseases, including autoimmune diseases, IBD, and potentially cancer[41-44]. Thus, these microorganisms are considered crucial for maintaining gut health and overall homeostasis throughout adulthood[45]. The beneficial effects of bifidobacteria on human gut health and their role in maintaining a balanced microbiota make them a primary focus in probiotic research. Their ability to modulate the gut microbiota, improve intestinal barrier function, and exert anti-inflammatory effects further underscores their importance as a key component in probiotic formulations. Numerous studies have demonstrated the efficacy of bifidobacteria in enhancing gut health, supporting immune function, and preventing gastrointestinal disorders[45-52].
In this review, we will delve into the specific roles and benefits of bifidobacteria as probiotic bacteria, exploring their mechanisms of action, clinical applications, and potential to promote gut health and overall well-being.
THE PROBIOTICS
The current definition, formulated in 2002 by the expert group of the Food and Agriculture Organization of the United Nations (FAO) and by World Health Organization (WHO), describes probiotics as “live microorganisms that, when administered in sufficient amounts, confer a health benefit on the host”[53]. This definition remains widely accepted within the scientific community. The International Scientific Association for Probiotics and Prebiotics (ISAPP) has endorsed this definition, emphasizing its flexibility in encompassing a broad range of products while maintaining key requirements such as viability and sufficient dosage. ISAPP also acknowledges the need for robust scientific evidence for strain-specific benefits, aligning with probiotic research’s evolving nature[54].
Probiotic microorganisms can be consumed naturally through foods, particularly fermented ones, but are also available as supplements or pharmaceutical products. Their integration into our diet can bring a series of benefits to our health. In fact, these beneficial microorganisms exert numerous beneficial effects on the human body, both at systemic and local levels, inhibiting the colonization of pathogenic bacteria[55], reducing intestinal inflammation[56], modulating the host’s immune response[57,58], and promoting intestinal eubiosis[59,60]. Additionally, probiotics exhibit a range of other health-promoting effects, including anti-diabetic, antioxidant, anti-aging, antimicrobial, and anti-biofilm properties[61-63]. The growing evidence of their health-promoting roles has prompted further investigations into their effects on specific pathologies, including gastrointestinal disorders/diseases, such as IBS, IBD, and antibiotic-associated diarrhea[64-67], suggesting the primary role of probiotic bacteria in the mechanisms involved in restoring the balance of the intestinal microbiota and reducing inflammation[68-71].
Despite the multiple health benefits, research on probiotics has highlighted some limitations, including the poorly understood molecular mechanisms, strain-specific behaviors, short-term effects, niche-specificity (both allochthonous and autochthonous), and the potential transfer of antibiotic resistance genes carried by probiotic bacteria to other members of the human microbiota[72,73]. Legislative regulations for the use of probiotics have been introduced to ensure consumer safety. For example, in the United States, microorganisms intended for probiotic products must obtain Generally Recognized As Safe (GRAS) status, regulated by the Food and Drug Administration (FDA). Similarly, in Europe, the European Food Safety Authority (EFSA) has established the concept of Qualified Presumption of Safety (QPS). QPS is a safety assessment approach that adds specific criteria to evaluate bacterial supplements. These criteria include verifying a history of safe use and confirming the absence of traits that could pose safety concerns, such as the potential for transferring antibiotic resistance genes[74-76]. However, the current regulation of probiotic bacteria varies significantly from country to country, and no common worldwide regulation exists.
The main probiotic microorganisms known and utilized in supplement products are mainly represented by species belonging to the Bifidobacterium and Lactobacillus genera, as well as other lactic acid bacteria, such as species belonging to Lactococcus and Streptococcus genera, but also Escherichia coli (E. coli) Nissle 1917, Enterococcus faecium, Bacillus coagulans, and the yeast Saccharomyces boulardii[77-79].
Interestingly, a new frontier of novel-generation probiotics has emerged to obtain the benefits of probiotic organisms without relying on their live forms. The term postbiotics has been broadly defined as preparations containing inanimate microbial cells and/or their metabolites that confer a health benefit on the host[80]. This definition includes both inactivated cells and the bioactive molecules they produce, such as enzymes, secreted proteins, short-chain fatty acids (SCFAs), vitamins, biosurfactants, amino acids, and peptides. However, in the very recent scientific literature, a distinction has begun to emerge between postbiotics and parabiotics[63,81,82], with the latter term being used to specifically refer to inactivated microbial cells or their crude extracts, while postbiotics now commonly refers to metabolic products or cell components. Although the term parabiotics is not yet fully recognized across the scientific community, it reflects an evolving understanding of these innovative approaches and their potential health benefits, complementing traditional probiotic use. Nevertheless, probiotics remain the most widely used supplements, particularly due to the strong evidence supporting their beneficial effects.
This review article provides readers with a detailed understanding of bifidobacteria and their potential to improve human health[83]. Bifidobacteria, including Bifidobacterium bifidum (B. bifidum), Bifidobacterium longum (B. longum), Bifidobacterium lactis (B. lactis) [formerly known as Bifidobacterium animalis
THERAPEUTIC POTENTIAL OF BIFIDOBACTERIAL SPECIES
Based on current scientific literature, which includes well-designed clinical studies and systematic reviews, it is clear that probiotics offer benefits to many different human body sites. The studies discussed in this section were selected through a detailed search of the currently existing scientific literature by using specific keywords such as the names of individual Bifidobacterium species, “probiotics”, and specific diseases or conditions. This approach ensured a comprehensive selection of relevant scientific literature on this topic. The team’s domain knowledge and expertise were also crucial in interpreting the findings and finalizing the inclusion of the most scientifically robust studies. The following section will examine studies on various species of the Bifidobacterium genus and their roles in specific diseases affecting major human body compartments, such as the gastrointestinal tract, respiratory tract, skeletal and muscular system, and nervous system [Table 1].
Bifidobacterial species discussed in this review with reported beneficial effects on specific diseases or disorders
PMID | Study type | Population characteristics | Bifidobacterial species | |
Constipation | 35079761 | Human | Adults | B. bifidum |
33996367 | Human | Adults | B. bifidum | |
33750988 | Animal | Murine | B. bifidum | |
36382178 | Human | Adults | B. longum | |
28884754 | Animal | Murine | B. longum | |
Antibiotic-associated diarrhea | 36558391 | Animal | Murine | B. bifidum |
31544979 | Animal | Murine | B. bifidum | |
35031969 | Human | Adults | B. lactis | |
34444974 | Human | Adults | B. lactis | |
Acute gastroenteritis-induced diarrhea | 26801008 | In vitro | - | B. adolescentis |
27375585 | In vitro | - | B. adolescentis | |
28432676 | In vitro | - | B. adolescentis | |
Stress-induced diarrhea | 23200466 | Animal | Murine | B. bifidum |
25918671 | Human | Adults | B. bifidum | |
IBS/IBD | 35935215 | Animal | Murine | B. bifidum |
21418261 | Human | Adults | B. bifidum | |
37240476 | Human | Adults | B. bifidum | |
32277872 | Human | Adults | B. bifidum | |
37702965 | Human | Adults | B. longum | |
21525768 | Human | Adults | B. breve | |
Helicobacter pylori infection | 29573807 | Human | Adults | B. bifidum |
Celiac disease | 21651295 | In vitro | - | B. longum |
18980693 | Human | Children | B. longum | |
26134988 | Human | Children | B. breve | |
27782071 | Human | Children and Adults | B. breve | |
Obesity, hypercholesterolemia, diabetes | 24985000 | Human | Children | B. bifidum |
23872958 | Animal | Murine | B. bifidum | |
25863679 | Animal and in vitro | Murine | B. bifidum | |
34365978 | Human | Adults | B. adolescentis | |
35745208 | Animal | Murine | B. adolescentis | |
21914236 | Animal | Murine | B. adolescentis | |
26090097 | Human | Adults | B. breve | |
30094122 | Human | Adults | B. breve | |
Respiratory infections and asthma | 26372517 | Human | Children | B.lactis |
36004715 | Human | Children | B.lactis | |
26840903 | Human | Adults | B. adolescentis | |
29633635 | Animal | Murine | B. adolescentis | |
Arthritis | 31168650 | Animal | Murine | B. bifidum, B. longum and B. breve |
32383727 | Animal | Murine | B. adolescentis | |
18848647 | Human | Adults | B. adolescentis | |
17953607 | Human | Adults | B. adolescentis | |
36377740 | Animal | Murine | B.breve | |
Anxiety and depression | 27801892 | Human | Adults | B.longum |
32300799 | Human | Adults | B.longum | |
37513541 | Human | Adults | B.longum | |
21988661 | Animal and in vitro | Murine | B.longum | |
32485204 | Animal | Murine | B.longum | |
32839473 | Animal and in vitro | Murine | B. adolescentis |
GASTROINTESTINAL TRACT
The gastrointestinal tract plays a key role in critical bodily functions, including nutrient absorption, digestion, and immune system regulation. Moreover, it is often affected by a range of diseases and disorders due to a combination of genetic predispositions and environmental factors. These altered conditions include, for example, constipation, antibiotic-associated diarrhea, gastroenteritis, IBS, IBD, Helicobacter pylori (H. pylori) infections, celiac disease, obesity, and diabetes. Extensive research efforts have demonstrated that Bifidobacterium-based probiotics can provide significant benefits in managing and alleviating symptoms associated with these gastrointestinal conditions.
Constipation
Constipation is a common and significant health concern, commonly described as reduced and/or difficult bowel movements[96].
Numerous studies have emphasized the potential of bifidobacteria as an effective treatment for constipation due to their beneficial impact on regulating intestinal microbiota, improving intestinal motility, and modulating inflammatory responses.
In this context, a human study demonstrated that the intake of B. bifidum leads to significant improvements in stool consistency and increased frequency of spontaneous bowel movements in individuals suffering from chronic constipation[97]. These effects are associated with the enhancement of the Firmicutes/Bacteroidetes ratio in the intestinal microbiota and increased concentrations of acetic and butyric acid[97]. These combined effects seem to lead to a notable improvement in clinical symptoms associated with functional constipation. Furthermore, the consumption of species belonging to the Bifidobacterium genus has been shown to significantly alter gut microbial metabolism, particularly influencing carbohydrate metabolism pathways such as propanoate and butanoate through interactions with other gut microbes. While Bifidobacterium species themselves do not produce these metabolites, they may facilitate their production by other members of the gut microbiota[98]. However, further metagenomic research is needed to identify the metabolic pathways involved precisely[98]. Moreover, studies conducted on murine models have further confirmed the effectiveness of B. bifidum in treating constipation, improving several physiological parameters and, consequentially, intestinal health. Specifically, B. bifidum has been reported to promote gut microbiota homeostasis by influencing butyrate production through cross-feeding interactions with other butyrate-producing bacteria. Butyric acid, a metabolite produced by the gut microbiota, has been shown to promote serotonin (5-HT) production by increasing the expression of the serotonin-producing enzyme tryptophan hydroxylase-1 (TPH1) in the host. Additionally, B. bifidum has been shown to regulate neurotransmitter levels, such as dopamine and acetylcholine, by suppressing dopamine increases and preventing acetylcholine decreases. These results suggest that B. bifidum may contribute to alleviating dysbiosis, enhancing organic acid levels, and improving neurotransmission[99].
Similarly, B. longum has been studied for its ability to improve bowel movement frequency, highlighting the importance of a personalized treatment approach based on individual microbial profiles[100]. In a human study, the intake of B. longum was associated with an increase in bacterial genera belonging to the Clostridia class, which are crucial for producing butyrate and other SCFAs, contributing to increased bowel movement frequency[100]. Moreover, murine model studies have further demonstrated the effectiveness of B. longum in alleviating constipation through mechanisms such as the modulation of intestinal function and reduction of inflammation[101,102].
Antibiotic-associated diarrhea
Numerous recent studies have documented the impact of antibiotics on the composition and functionality of the microbiota[103-105]. Antibiotics, especially those with a broad spectrum, act on a wide range of microorganisms, including the normal microbiota of an individual, altering intestinal microbiota composition and particularly reducing its diversity. Consequently, antibiotics might disrupt host-microbe interactions, compromising immune system homeostasis and reducing resistance to colonization by pathogenic strains. Intestinal dysbiosis, defined as the disruption of the symbiotic balance between the microbiota and the host that antibiotics may induce, can, in turn, lead to diarrhea and recurrent infections caused by opportunistic pathogens such as Clostridioides difficile[106-109].
In murine models, it has been shown that probiotic therapy based on B. bifidum alleviates diarrhea symptoms, restores the structure of intestinal villi, and improves microbiota health. Additionally, it has been demonstrated that B. bifidum alleviates inflammation and tissue damage in sodium dextran sulfate-induced colitis in murine models[110]. Similarly, a specific murine model with diarrhea caused by E. coli overgrowth, simulating antibiotic-induced dysbiosis, demonstrated that the supplementation of B. bifidum improved dysbiosis and suppressed diarrhea symptoms by reducing the excessive growth of E. coli in the intestine[111]. These beneficial effects are probably also related to the increased production of IgA induced by B. bifidum, which easily binds to E. coli, reducing its growth and resolving diarrhea. Furthermore, the analysis of the microbiota composition has shown a trend toward an increase in butyrate-producing bacteria associated with the treatment involving B. bifidum. In fact, butyric acid, a SCFA, is used as an energy source by intestinal epithelial cells, contributing to regulating the intestinal epithelium and improving intestinal activity[111-113].
A recent study regarding B. lactis has demonstrated its effectiveness in preventing diarrhea and alleviating gastrointestinal symptoms in hospitalized patients undergoing antibiotic treatment, significantly reducing symptoms[114]. Moreover, it has been observed that consuming yogurt containing this species during antibiotic treatment mitigates the negative impact of antibiotics on fecal microbiota. This beneficial effect may be attributed to yogurt containing B. lactis, which attenuated the decrease in acetate levels following antibiotic treatment and facilitated a quicker return to baseline SCFA levels[115]. Furthermore, it has been shown in a murine model that supplementation of B. lactis during antibiotic-associated diarrhea led to significant improvement in symptoms, contributing to restoring intestinal microbiota and reducing inflammation[116].
Acute diarrhea caused by gastroenteritis
Acute gastroenteritis represents one of the major global health issues, particularly in children[117]. The primary etiological agents of gastroenteritis in children are Rotaviruses and Noroviruses[118-120], and the main symptoms include abdominal pain, profuse diarrhea, and vomiting. Although the primary treatment focuses on electrolyte replenishment and rehydration[121-123], the use of selected probiotic microorganisms may also be beneficial in alleviating the symptoms of the infection.
Bifidobacterium adolescentis (B. adolescentis), for example, exhibits potential antiviral effects against rotavirus and noroviruses. In fact, B. adolescentis metabolites could alter the replication of viral particles, specifically by reducing the intracellular amount of NSP4 and Ca2+ release, thereby decreasing the virus’s ability to enter cells and replicate[124-126]. Furthermore, protein metabolites obtained from B. adolescentis cells could prevent the entry of rotavirus by directly affecting the viral particle. The hypothesized mechanism involves the adhesion process to cellular receptors not being efficiently executed due to alterations in the viral outer proteins, such as VP7 or VP4. However, further studies are needed to elucidate this antiviral activity’s mechanisms, including direct interactions with cellular receptors or intracellular regulatory processes[127]. This probiotic microorganism has also exhibited antiviral effects against Coxsackievirus B3, reducing the number of viral sequence copies. The mechanism of action related to antiviral effects requires further study. Additionally, B. adolescentis has been shown to provide protection against intestinal bacterial infections by Yersinia enterocolitica in murine models, suggesting that the presence of B. adolescentis might offer protection against yersiniosis by enhancing epithelial barrier function through direct interactions with intestinal epithelial cells and by altering the composition of the microbiota[128,129].
In addition to antibiotic-associated diarrhea and diarrhea related to viral infections, several other forms of diarrhea are influenced by other various factors[130].
Among these forms, one of particular interest is stress-induced diarrhea. The brain-gut-enteric microbiota axis may modulate this effect, and it is bidirectional[131-133]. Moreover, several studies have demonstrated that B. bifidum effectively alleviates gastrointestinal disorders and reduces stress in university students, indicating its positive impact on both psychological and physical well-being[131]. Specifically, oral intake of
IBD
Numerous studies have examined the effectiveness of B. bifidum, B. breve, and B. longum in treating symptoms associated with IBD, such as bloating, gas, abdominal pain, cramps, and other digestive disorders[137-140]. IBD, which includes both Crohn’s disease (CD) and ulcerative colitis (UC), is of particular interest. IBD should be regarded as a systemic condition, not confined to the gastrointestinal tract alone, as many patients exhibit symptoms outside the intestine. While CD and UC share common pathological and clinical characteristics, each also presents specific distinctive differences[141]. These studies provide an overview of the intestinal health benefits these probiotics offer and the mechanisms of action involved.
Regarding B. bifidum, several studies have highlighted its role in improving quality of life and alleviating gastrointestinal symptoms in subjects with IBD. A recent murine clinical demonstrated that early treatment with B. bifidum can reduce inflammation and promote intestinal mucosal integrity, suggesting a potential protective effect against long-term colitis. The molecular mechanisms involved are not yet fully understood, but the production of specific metabolites, such as acetic and butyric SCFA, can promote the healthy development of the intestine, supporting the growth and health of the intestinal mucosa[142].
As for B. breve, several studies have highlighted its efficacy in improving clinical conditions in patients with UC. In detail, B. breve intake, either alone or in combination with galacto-oligosaccharides (GOS), led to significant improvements in clinical parameters and a rebalancing of the intestinal microbiota. Moreover,
IBS
Several studies have examined the effectiveness of Bifidobacterium-based probiotics in treating symptoms associated with IBS, highlighting their role in improving quality of life and alleviating gastrointestinal symptoms in affected individuals. Specifically, recent studies on B. bifidum have demonstrated that a four-week intake significantly reduces the severity index of IBS and improves symptoms such as abdominal pain and dyspepsia. Therefore, B. bifidum treatment has significantly alleviated these symptoms, although the underlying mechanisms remain largely unclear[144-146]. Moreover, the efficacy of B. longum in reducing IBS symptoms has been investigated, focusing primarily on bloating, abdominal pain, and constipation. The results highlighted that an eight-week intake of B. longum significantly reduces IBS symptoms[147]. An additional clinical trial confirmed that B. longum could improve the quality of life and reduce the severity of IBS in affected patients. However, further research still needs to clarify various mechanisms through which B. longum exerts these benefits[148].
Mitigation of the effects of H. pylori infection
H. pylori is a Gram-negative, opportunistic pathogen that colonizes the human stomach and is implicated in various gastrointestinal diseases. In fact, H. pylori infection can lead to gastric and duodenal ulcers, gastritis, and gastric carcinoma. Eradication of the bacterium through antibiotic therapy causes an alteration of the intestinal microbiota, which can be mitigated with the use of probiotics. Furthermore, probiotics offer additional benefits in this clinical context, extending beyond merely mitigating effects on the microbiota[149].
Supplementation with B. bifidum has been shown to alleviate infection symptoms in healthy adults effectively. Significant relief from postprandial discomfort and epigastric pain has been reported after consuming fermented milk encompassing B. bifidum cells for four weeks[150]. B. bifidum has been found to enhance the physical gastric barrier and regulate NF-kB signaling in conditions such as H. pylori-associated gastritis, providing new insights into the treatment of gastroesophageal reflux disease and related disorders[151]. Additionally, consuming fermented milk containing B. bifidum has been reported to improve gastrointestinal symptoms and reduce stress markers in subjects with functional gastrointestinal disorders[136].
Moreover, it has been highlighted that B. lactis aids in suppressing H. pylori, decreasing bacterial load and gastric mucosal damage in infected gerbils, and lowering inflammatory cytokine levels. These findings suggest that B. lactis could be a complementary approach in H. pylori infection management[152]. Moreover, a significant improvement in symptoms has been observed in women with digestive symptoms such as abdominal pain or discomfort, bloating, flatulence, and stomach rumbling after the intake for two weeks of a fermented milk product containing B. lactis[153].
Celiac disease
Celiac disease is an autoimmune disease affecting the small intestine. Since gluten is the triggering factor, the only current treatment is a strict gluten-free diet. In recent years, a correlation between intestinal dysbiosis and celiac disease has been observed, leading to the exploration of complementary therapeutic strategies. Among these, modulation of the intestinal microbiota has emerged as a promising area of research[154].
B. breve has been investigated for its role in the treatment of celiac disease and food intolerances, and it has been reported that this probiotic bacterium can improve the symptoms of celiac disease by modulating the immune response and altering the fecal microbiota[155]. Moreover, several studies demonstrate that B. breve strains, in combination with a gluten-free diet (GFD), temporarily reduce TNF-α production in children with celiac disease, thereby counteracting the pro-inflammatory environment. Reducing TNF-α could decrease the intestinal and systemic complications of celiac disease. Further studies are needed to confirm the benefit of this probiotic as a complementary therapy to a GFD[156,157].
Similarly, B. longum has shown potential benefits in the treatment of celiac disease. In this context, it has been observed that B. longum can reduce gliadin toxicity and modify the response of intestinal cells, suggesting a possible protective role against celiac disease[158]. Further studies have confirmed the efficacy of B. longum in modulating the immune response, reducing pro-inflammatory cytokines, and improving gluten tolerance in celiac disease models[159,160].
Obesity, hypercholesterolemia and diabetes
The gastrointestinal tract plays a crucial role in overall metabolic health, influencing conditions such as obesity, hypercholesterolemia, and diabetes. These metabolic disorders are interconnected with gut microbiota composition, where probiotics, particularly those based on Bifidobacterium species, have shown promising therapeutic potential.
In this context, it has been demonstrated that administering B. bifidum to children with primary dyslipidemia significantly improved total cholesterol and low-density lipoprotein cholesterol (LDL-C) levels[161]. Another study investigated 34 human strains of the Bifidobacterium genus, which were assessed for their cholesterol adsorption capacity and bile salt hydrolase activity, which represent two strain-specific characteristics. It was found that two strains of the species B. bifidum exhibited a significant ability to adsorb cholesterol. The administration of a probiotic formulation containing these strains led to a significant reduction in total cholesterol and LDL-C levels. However, no effects were observed on high-density lipoprotein cholesterol (HDL-C) levels or the HDL-C/LDL-C ratio[162]. Similarly, another study revealed the high capacity of B. bifidum to assimilate cholesterol, contributing to the reduction of total cholesterol and LDL-C in murine models, and indicated the capability of a specific strain, i.e., B. bifidum PRL2010, to reduce cholesterol by converting it into coprostanol, with possible beneficial effects on cardiovascular health[163].
Regarding B. breve, significant fat mass reductions and blood parameters improvements related to liver function and inflammation were observed in adults prone to obesity. In detail, several studies suggest that
Furthermore, the beneficial effect of the combination of berberine and B. adolescentis has been highlighted in a recent study, which demonstrated a significant reduction in fasting blood glucose levels and glycated hemoglobin, along with an improvement in the composition of the intestinal microbiota in subjects with hyperglycemia[167]. Moreover, Bifidobacterium strains seemed to alleviate type 2 diabetes symptoms in mice, reducing inflammation and increasing acetic acid and butyric acid levels. These SCFAs enhance energy homeostasis and may mitigate metabolic disorders. Supplementation with B. adolescentis or B. bifidum significantly increased SCFA levels and reduced blood glucose levels. Furthermore, SCFAs were negatively correlated with glucose, insulin resistance, and inflammatory markers, suggesting that the beneficial effects of these strains are linked to their impact on SCFAs[168]. Further research has observed a reduction in visceral fat accumulation and inflammation in murine models, suggesting a potential benefit of B. adolescentis in the management of obesity and diabetes[169,170].
RESPIRATORY TRACT
Historically, the respiratory tract was considered a sterile environment. However, recent studies have revealed a diverse microbial community residing in the respiratory tract, which appears to play a significant role in maintaining human health. Growing scientific evidence suggests a relationship between respiratory microbiota composition and overall respiratory health. Additionally, the gut-lung axis is a concept that describes the bidirectional interaction between the gut and respiratory microbiota. This interaction suggests that probiotics positively affect gut microbiota and may also influence respiratory health by modulating immune responses and microbial communities across these two systems.
Respiratory tract infections and asthma
Respiratory tract infections and asthma are common conditions that significantly impact global health. Emerging research has shown that probiotics, particularly species from the genus Bifidobacterium, may play a beneficial role in managing these conditions.
In this context, a recent study regarding children with respiratory tract infections demonstrated that administering probiotic products containing B. lactis twice daily could reduce respiratory infection incidence[171]. Moreover, another study explored the effect of controlled administration of B. lactis on an infant cohort, observing a decrease in respiratory tract infections during the first eight months of life in those treated with the probiotic compared to the control group[172]. Additionally, a randomized, double-blind, placebo-controlled trial assessed the benefits of probiotics in infant formula on upper respiratory tract infections (URTIs) in infants aged six to 15 months. The study examined the effect of the probiotic B. lactis on reducing URTIs. The results showed that no infants who received the probiotic developed URTIs or required antibiotic or antiviral treatments, unlike the control group, which experienced significant infection rates, demonstrating a significant reduction in URTIs in the probiotic-treated group[173]. Furthermore,
SKELETAL AND MUSCULAR SYSTEM
The skeletal and muscular systems are fundamental to human movement and structural integrity. They are often affected by several conditions, such as arthritis, significantly impacting quality of life. Recent studies have explored the potential benefits of probiotics, particularly Bifidobacterium species, in managing these conditions by modulating the gut microbiota and immune response.
Arthritis
Arthritis, a chronic inflammatory condition affecting the joints, can severely impact mobility and quality of life. Increasing scientific interest has focused on the potential therapeutic benefits of probiotics, particularly Bifidobacterium strains, in managing arthritis. These probiotic bacteria may modulate the immune response and intestinal microbiota, offering new perspectives in the treatment of rheumatoid arthritis (RA) and other forms of arthritis. In particular, the treatment of arthritis through the use of probiotic strains such as
Furthermore, increasing evidence suggests a significant correlation between the development of RA and the periodontal disease, characterized by the presence of Porphyromonas gingivalis (P. gingivalis). The higher frequency of antibodies against P. gingivalis in patients with RA indicates a potential involvement of this bacterium in the disease’s pathogenesis. In this context, the administration of B. adolescentis may be beneficial, as it reduces the concentration of vitamin K, a nutrient essential for the survival and virulence of P. gingivalis, thereby potentially mitigating its impact on both oral and intestinal microbiota and contributing to the management of RA[179-182].
Similarly, B. breve has shown efficacy in repairing the intestinal barrier and reducing systemic inflammation in collagen-induced arthritis murine models[183]. This probiotic positively influenced intestinal microbiota composition, increased SCFA levels, and inhibited inflammatory pathways, thereby improving conditions such as arthritis. In a study of arthritic rats, evaluation of serum markers of arthritis, such as C-reactive protein (CRP) and rheumatoid factor (RF), showed that the group treated with B. breve had significantly reduced levels of these markers compared to the untreated control rats. Moreover, the administration of
NERVOUS SYSTEM
The nervous system, encompassing the brain and spinal cord, regulates and coordinates body activities. Emerging research has proposed the existence of a gut-brain axis, a bidirectional communication pathway between the gut microbiota and the brain, suggesting that gut health can significantly impact mental health. This concept underpins the potential of probiotics to influence neurological conditions.
Anxiety and depression
Anxiety and depression are increasingly common mental health disorders that can significantly impair daily functioning and quality of life[185]. These conditions are often associated with gastrointestinal issues, highlighting a potential link between gut health and mental well-being[186,187]. In this context, the new concept of the gut-brain axis suggests that gut microbiota can influence brain function and mental health, providing a rationale for exploring the therapeutic potential of probiotics in managing anxiety and depression[186,188].
Recent studies have revealed that the gut microbiota can synthesize GABA, i.e., γ-Aminobutyric acid, a key inhibitory neurotransmitter in the regulation of the gut-brain axis[189,190]. Alterations in GABA metabolism have been associated with disorders such as anxiety and depression[190-192]. A recent genomic analysis conducted on over 1,000 bifidobacteria strains identified B. adolescentis as a promising producer of GABA in the human gastrointestinal tract[94]. Furthermore, in silico analysis of metagenomic data from human and animal studies showed a possible correlation between the presence of B. adolescentis and mental disorders, such as depression and anxiety. Moreover, in vivo experiments using the murine model further supported these findings. In fact, specific strains of B. adolescentis, administered to rats, demonstrated the ability to increase GABA production. These results suggest that B. adolescentis could play a significant role in gut-brain axis interactions and contribute to developing new therapeutic strategies for mental disorders by modulating the gut microbiota[94].
Recent research on the efficacy of B. longum in the treatment of anxiety and depression has yielded promising results, highlighting the potential of these probiotics to modulate key aspects of mental health. In detail, B. longum has been demonstrated to possess the ability to mitigate stress response and promote cognitive improvements, reducing cortisol production and enhancing visuospatial memory[193]. Similarly, it has been shown that B. longum improved mental flexibility and reduced stress in the elderly, suggesting a positive impact on their mental well-being[194]. Furthermore, another study described that supplementation with B. longum significantly reduces perceived stress and improves sleep quality, highlighting the role of this probiotic bacteria in enhancing stress management and overall mental health[195]. Additional research efforts have been made to explore the physiological and behavioral effects of B. longum, revealing that this probiotic microorganism modifies neural oscillations in response to social stress, suggesting an impact on brain functions associated with stress management[196]. Moreover, further studies have elucidated the mechanism through which B. longum influences anxious behavior and the functioning of the hypothalamic-pituitary-adrenal axis, providing a basis for understanding how probiotics can directly influence stress-related behavior and physiology[197,198].
CONCLUSION
Bifidobacteria, as probiotics, offer substantial therapeutic benefits across various health conditions by modulating the intestinal microbiota and immune responses[58,199]. A significant proportion of clinical studies on probiotics focus on bifidobacteria, highlighting their essential role in human health[200,201].
Bifidobacteria have co-evolved with humans and are among the first microorganisms colonizing the infant’s gut and playing a crucial role in the early development of the intestinal microbiota[11]. They are essential for educating the immune system and supporting gut physiology, such as stimulating mucin production[92,202]. Additionally, bifidobacteria are maintained in the human gut due to the production of prebiotic molecules like mucin[36,38], which support their survival. Moreover, recent studies have reported the transmission of these bacteria from mother to child through vertical transmission mechanisms[40], again underscoring their co-evolution with the human host. The loss of bifidobacteria in both children and adults has been negatively correlated with numerous human diseases and disorders[51,138,200], further emphasizing their critical role in maintaining gut health. In this context, the beneficial effects of bifidobacteria in the gastrointestinal tract are well documented.
In summary, bifidobacteria are fundamental to human health, having co-evolved with their host and playing a critical role from early life. Their ability to modulate the gut microbiota, support immune function, and maintain intestinal barrier integrity makes them indispensable in promoting health and preventing disease. This review underscores the significant therapeutic potential of Bifidobacterium species across various body compartments and highlights the necessity for further research to understand the underlying mechanisms and validate clinical efficacy. Additionally, more studies are necessary to identify the genetic bases underlying these beneficial effects and to better understand the interactions with the host.
DECLARATIONS
Authors’ contributions
Drafted manuscript: Bocchio F, Mancabelli L, Milani C
Figure editing: Bocchio F, Lugli GA, Tarracchini C, Longhi G
Edited and revised manuscript: De Conto F, Turroni F, Ventura M
Provided funding: Turroni F, Ventura M
Availability of data and materials
Not applicable.
Financial support and sponsorship
This study was supported by “Programma Operativo Nazionale Ricerca e Innovazione” 2014-2020 (PON “R&I” 2014-2020) (project ARS01_00530). FT was supported by PROGETTO Ricerca Finalizzata, Ministero della Salute (RF GR-2018-12365988).
Conflicts of interest
Mancabelli L, Lugli GA, and Turroni F are Editorial Board members of Microbiome Research Reports. Ventura M is the Editor-in-Chief of the journal Microbiome Research Reports. Other authors declared that there are no conflicts of interest.
Ethical approval and consent to participate
Not applicable.
Consent for publication
Not applicable.
Copyright
© The Author(s) 2024.
REFERENCES
1. Fan Y, Pedersen O. Gut microbiota in human metabolic health and disease. Nat Rev Microbiol 2021;19:55-71.
2. Lynch SV, Pedersen O. The human intestinal microbiome in health and disease. N Engl J Med 2016;375:2369-79.
3. Candela M, Perna F, Carnevali P, et al. Interaction of probiotic Lactobacillus and Bifidobacterium strains with human intestinal epithelial cells: adhesion properties, competition against enteropathogens and modulation of IL-8 production. Int J Food Microbiol 2008;125:286-92.
4. Olszak T, An D, Zeissig S, et al. Microbial exposure during early life has persistent effects on natural killer T cell function. Science 2012;336:489-93.
5. Fukuda S, Toh H, Hase K, et al. Bifidobacteria can protect from enteropathogenic infection through production of acetate. Nature 2011;469:543-7.
6. Cullin N, Azevedo Antunes C, Straussman R, Stein-Thoeringer CK, Elinav E. Microbiome and cancer. Cancer Cell 2021;39:1317-41.
7. Reynoso-García J, Miranda-Santiago AE, Meléndez-Vázquez NM, et al. A complete guide to human microbiomes: body niches, transmission, development, dysbiosis, and restoration. Front Syst Biol 2022;2:951403.
8. Li N, Zuo B, Huang S, et al. Spatial heterogeneity of bacterial colonization across different gut segments following inter-species microbiota transplantation. Microbiome 2020;8:161.
9. Gomaa EZ. Human gut microbiota/microbiome in health and diseases: a review. Antonie Van Leeuwenhoek 2020;113:2019-40.
10. Carding S, Verbeke K, Vipond DT, Corfe BM, Owen LJ. Dysbiosis of the gut microbiota in disease. Microb Ecol Health Dis 2015;26:26191.
11. Milani C, Duranti S, Bottacini F, et al. The first microbial colonizers of the human gut: composition, activities, and health implications of the infant gut microbiota. Microbiol Mol Biol Rev 2017;81:e00036-17.
12. Fassarella M, Blaak EE, Penders J, Nauta A, Smidt H, Zoetendal EG. Gut microbiome stability and resilience: elucidating the response to perturbations in order to modulate gut health. Gut 2021;70:595-605.
13. Mancabelli L, Milani C, Fontana F, et al. A pilot study to disentangle the infant gut microbiota composition and identification of bacteria correlates with high fat mass. Microbiome Res Rep 2023;2:23.
14. Requena T, Velasco M. The human microbiome in sickness and in health. Rev Clin Esp 2021;221:233-40.
16. Baumgart DC, Carding SR. Inflammatory bowel disease: cause and immunobiology. Lancet 2007;369:1627-40.
17. De Palma G, Nadal I, Medina M, et al. Intestinal dysbiosis and reduced immunoglobulin-coated bacteria associated with coeliac disease in children. BMC Microbiol 2010;10:63.
18. Guinane CM, Cotter PD. Role of the gut microbiota in health and chronic gastrointestinal disease: understanding a hidden metabolic organ. Therap Adv Gastroenterol 2013;6:295-308.
19. Perler BK, Friedman ES, Wu GD. The role of the gut microbiota in the relationship between diet and human health. Annu Rev Physiol 2023;85:449-68.
20. Yadav MK, Kumari I, Singh B, Sharma KK, Tiwari SK. Probiotics, prebiotics and synbiotics: Safe options for next-generation therapeutics. Appl Microbiol Biotechnol 2022;106:505-21.
21. Gibson GR, Roberfroid MB. Dietary modulation of the human colonic microbiota: introducing the concept of prebiotics. J Nutr 1995;125:1401-12.
22. Bakker GJ, Nieuwdorp M. Fecal microbiota transplantation: therapeutic potential for a multitude of diseases beyond clostridium difficile. Microbiol Spectr 2017;5:291-308.
23. Evrensel A, Ceylan ME. Fecal microbiota transplantation and its usage in neuropsychiatric disorders. Clin Psychopharmacol Neurosci 2016;14:231-7.
24. Ramai D, Zakhia K, Ofosu A, Ofori E, Reddy M. Fecal microbiota transplantation: donor relation, fresh or frozen, delivery methods, cost-effectiveness. Ann Gastroenterol 2019;32:30-8.
25. Rossen NG, MacDonald JK, de Vries EM, et al. Fecal microbiota transplantation as novel therapy in gastroenterology: a systematic review. World J Gastroenterol 2015;21:5359-71.
26. Vendrik KEW, Ooijevaar RE, de Jong PRC, et al. Fecal microbiota transplantation in neurological disorders. Front Cell Infect Microbiol 2020;10:98.
27. Xu HM, Huang HL, Zhou YL, et al. Fecal microbiota transplantation: a new therapeutic attempt from the gut to the brain. Gastroenterol Res Pract 2021;2021:6699268.
28. Biazzo M, Deidda G. Fecal microbiota transplantation as new therapeutic avenue for human diseases. J Clin Med 2022;11:4119.
29. Ooijevaar RE, Terveer EM, Verspaget HW, Kuijper EJ, Keller JJ. Clinical application and potential of fecal microbiota transplantation. Annu Rev Med 2019;70:335-51.
30. Wang JW, Kuo CH, Kuo FC, et al. Fecal microbiota transplantation: review and update. J Formos Med Assoc 2019;118:S23-31.
32. Linares DM, Gómez C, Renes E, et al. Lactic acid bacteria and bifidobacteria with potential to design natural biofunctional health-promoting dairy foods. Front Microbiol 2017;8:846.
33. Piqué N, Berlanga M, Miñana-Galbis D. Health benefits of heat-killed (tyndallized) probiotics: an overview. Int J Mol Sci 2019;20:2534.
34. Dominguez-Bello MG, Costello EK, Contreras M, et al. Delivery mode shapes the acquisition and structure of the initial microbiota across multiple body habitats in newborns. Proc Natl Acad Sci U S A 2010;107:11971-5.
35. Cukrowska B, Bierła JB, Zakrzewska M, Klukowski M, Maciorkowska E. The relationship between the infant gut microbiota and allergy. The role of Bifidobacterium breve and prebiotic oligosaccharides in the activation of anti-allergic mechanisms in early life. Nutrients 2020;12:946.
36. Turroni F, Duranti S, Bottacini F, Guglielmetti S, Van Sinderen D, Ventura M. Bifidobacterium bifidum as an example of a specialized human gut commensal. Front Microbiol 2014;5:437.
37. Gutierrez A, Pucket B, Engevik MA. Bifidobacterium and the intestinal mucus layer. Microbiome Res Rep 2023;2:36.
38. Ruas-Madiedo P, Gueimonde M, Fernández-García M, de los Reyes-Gavilán CG, Margolles A. Mucin degradation by Bifidobacterium strains isolated from the human intestinal microbiota. Appl Environ Microbiol 2008;74:1936-40.
39. Gavzy SJ, Kensiski A, Lee ZL, Mongodin EF, Ma B, Bromberg JS. Bifidobacterium mechanisms of immune modulation and tolerance. Gut Microbes 2023;15:2291164.
40. Milani C, Mancabelli L, Lugli GA, et al. Exploring vertical transmission of bifidobacteria from mother to child. Appl Environ Microbiol 2015;81:7078-87.
41. Favier C, Neut C, Mizon C, Cortot A, Colombel JF, Mizon J. Fecal beta-D-galactosidase production and Bifidobacteria are decreased in Crohn’s disease. Dig Dis Sci 1997;42:817-22.
42. Macfarlane S, Furrie E, Cummings JH, Macfarlane GT. Chemotaxonomic analysis of bacterial populations colonizing the rectal mucosa in patients with ulcerative colitis. Clin Infect Dis 2004;38:1690-9.
43. Mylonaki M, Rayment NB, Rampton DS, Hudspith BN, Brostoff J. Molecular characterization of rectal mucosa-associated bacterial flora in inflammatory bowel disease. Inflamm Bowel Dis 2005;11:481-7.
44. Gueimonde M, Ouwehand A, Huhtinen H, Salminen E, Salminen S. Qualitative and quantitative analyses of the bifidobacterial microbiota in the colonic mucosa of patients with colorectal cancer, diverticulitis and inflammatory bowel disease. World J Gastroenterol 2007;13:3985-9.
45. Martin AJM, Serebrinsky-Duek K, Riquelme E, Saa PA, Garrido D. Microbial interactions and the homeostasis of the gut microbiome: the role of Bifidobacterium. Microbiome Res Rep 2023;2:17.
46. Kiseleva EP, Mikhailopulo KI, Sviridov OV, Novik GI, Knirel YA, Szwajcer Dey E. The role of components of Bifidobacterium and Lactobacillus in pathogenesis and serologic diagnosis of autoimmune thyroid diseases. Benef Microbes 2011;2:139-54.
47. Benvenga S, Guarneri F. Molecular mimicry and autoimmune thyroid disease. Rev Endocr Metab Disord 2016;17:485-98.
48. López P, González-Rodríguez I, Sánchez B, et al. Interaction of Bifidobacterium bifidum LMG13195 with HT29 cells influences regulatory-T-cell-associated chemokine receptor expression. Appl Environ Microbiol 2012;78:2850-7.
49. Agrawal A, Houghton LA, Morris J, et al. Clinical trial: the effects of a fermented milk product containing Bifidobacterium lactis DN-173 010 on abdominal distension and gastrointestinal transit in irritable bowel syndrome with constipation. Aliment Pharmacol Ther 2009;29:104-14.
50. Guyonnet D, Chassany O, Ducrotte P, et al. Effect of a fermented milk containing Bifidobacterium animalis DN-173 010 on the health-related quality of life and symptoms in irritable bowel syndrome in adults in primary care: a multicentre, randomized, double-blind, controlled trial. Aliment Pharmacol Ther 2007;26:475-86.
51. Hidalgo-Cantabrana C, Delgado S, Ruiz L, Ruas-Madiedo P, Sánchez B, Margolles A. Bifidobacteria and their health-promoting effects. Microbiol Spectr 2017;5:73-98.
52. Muñoz JA, Chenoll E, Casinos B, et al. Novel probiotic Bifidobacterium longum subsp. infantis CECT 7210 strain active against rotavirus infections. Appl Environ Microbiol 2011;77:8775-83.
53. Hill C, Guarner F, Reid G, et al. Expert consensus document. The International Scientific Association for Probiotics and Prebiotics consensus statement on the scope and appropriate use of the term probiotic. Nat Rev Gastroenterol Hepatol 2014;11:506-14.
54. Gibson GR, Hutkins R, Sanders ME, et al. Expert consensus document: The International Scientific Association for Probiotics and Prebiotics (ISAPP) consensus statement on the definition and scope of prebiotics. Nat Rev Gastroenterol Hepatol 2017;14:491-502.
55. Rahman MN, Barua N, Tin MCF, Dharmaratne P, Wong SH, Ip M. The use of probiotics and prebiotics in decolonizing pathogenic bacteria from the gut; a systematic review and meta-analysis of clinical outcomes. Gut Microbes 2024;16:2356279.
56. Li C, Peng K, Xiao S, Long Y, Yu Q. The role of Lactobacillus in inflammatory bowel disease: from actualities to prospects. Cell Death Discov 2023;9:361.
57. Mousa WK, Mousa S, Ghemrawi R, et al. Probiotics modulate host immune response and interact with the gut microbiota: shaping their composition and mediating antibiotic resistance. Int J Mol Sci 2023;24:13783.
58. Liu Y, Wang J, Wu C. Modulation of gut microbiota and immune system by probiotics, pre-biotics, and post-biotics. Front Nutr 2021;8:634897.
59. Hemarajata P, Versalovic J. Effects of probiotics on gut microbiota: mechanisms of intestinal immunomodulation and neuromodulation. Therap Adv Gastroenterol 2013;6:39-51.
60. Petrariu OA, Barbu IC, Niculescu AG, et al. Role of probiotics in managing various human diseases, from oral pathology to cancer and gastrointestinal diseases. Front Microbiol 2023;14:1296447.
61. Galdeano C, Cazorla SI, Lemme Dumit JM, Vélez E, Perdigón G. Beneficial effects of probiotic consumption on the immune system. Ann Nutr Metab 2019;74:115-24.
62. Majeed M, Nagabhushanam K, Arumugam S, Majeed S, Ali F. Bacillus coagulans MTCC 5856 for the management of major depression with irritable bowel syndrome: a randomised, double-blind, placebo controlled, multi-centre, pilot clinical study. Food Nutr Res 2018;62.
63. Nataraj BH, Ali SA, Behare PV, Yadav H. Postbiotics-parabiotics: the new horizons in microbial biotherapy and functional foods. Microb Cell Fact 2020;19:168.
64. Martyniak A, Medyńska-Przęczek A, Wędrychowicz A, Skoczeń S, Tomasik PJ. Prebiotics, probiotics, synbiotics, paraprobiotics and postbiotic compounds in IBD. Biomolecules 2021;11:1903.
65. Ford AC, Quigley EM, Lacy BE, et al. Efficacy of prebiotics, probiotics, and synbiotics in irritable bowel syndrome and chronic idiopathic constipation: systematic review and meta-analysis. Am J Gastroenterol 2014;109:1547-61.
66. Li B, Liang L, Deng H, Guo J, Shu H, Zhang L. Efficacy and safety of probiotics in irritable bowel syndrome: a systematic review and meta-analysis. Front Pharmacol 2020;11:332.
67. Goodman C, Keating G, Georgousopoulou E, Hespe C, Levett K. Probiotics for the prevention of antibiotic-associated diarrhoea: a systematic review and meta-analysis. BMJ Open 2021;11:e043054.
68. Mazziotta C, Tognon M, Martini F, Torreggiani E, Rotondo JC. Probiotics mechanism of action on immune cells and beneficial effects on human health. Cells 2023;12:184.
69. Kopacz K, Phadtare S. Probiotics for the prevention of antibiotic-associated diarrhea. Healthcare 2022;10:1450.
70. Cremon C, Barbaro MR, Ventura M, Barbara G. Pre- and probiotic overview. Curr Opin Pharmacol 2018;43:87-92.
71. Roy S, Dhaneshwar S. Role of prebiotics, probiotics, and synbiotics in management of inflammatory bowel disease: current perspectives. World J Gastroenterol 2023;29:2078-100.
72. Suez J, Zmora N, Segal E, Elinav E. The pros, cons, and many unknowns of probiotics. Nat Med 2019;25:716-29.
73. Evivie SE, Huo GC, Igene JO, Bian X. Some current applications, limitations and future perspectives of lactic acid bacteria as probiotics. Food Nutr Res 2017;61:1318034.
74. Anadón A, Martínez-Larrañaga MR, Aranzazu Martínez M. Probiotics for animal nutrition in the European Union. Regulation and safety assessment. Regul Toxicol Pharmacol 2006;45:91-5.
75. Gaggìa F, Mattarelli P, Biavati B. Probiotics and prebiotics in animal feeding for safe food production. Int J Food Microbiol 2010;141:S15-28.
76. Markowiak P, Śliżewska K. Effects of probiotics, prebiotics, and synbiotics on human health. Nutrients 2017;9:1021.
77. Azad MAK, Sarker M, Li T, Yin J. Probiotic species in the modulation of gut microbiota: an overview. Biomed Res Int 2018;2018:9478630.
78. Holzapfel WH, Haberer P, Geisen R, Björkroth J, Schillinger U. Taxonomy and important features of probiotic microorganisms in food and nutrition. Am J Clin Nutr 2001;73:365S-73S.
79. Kechagia M, Basoulis D, Konstantopoulou S, et al. Health benefits of probiotics: a review. ISRN Nutr 2013;2013:481651.
80. Salminen S, Collado MC, Endo A, et al. The International Scientific Association of Probiotics and Prebiotics (ISAPP) consensus statement on the definition and scope of postbiotics. Nat Rev Gastroenterol Hepatol 2021;18:649-67.
81. Cuevas-González PF, Liceaga AM, Aguilar-Toalá JE. Postbiotics and paraprobiotics: from concepts to applications. Food Res Int 2020;136:109502.
82. Hosseini SH, Farhangfar A, Moradi M, Dalir-Naghadeh B. Beyond probiotics: exploring the potential of postbiotics and parabiotics in veterinary medicine. Res Vet Sci 2024;167:105133.
83. Chen J, Chen X, Ho CL. Recent development of probiotic bifidobacteria for treating human diseases. Front Bioeng Biotechnol 2021;9:770248.
84. Jäger R, Mohr AE, Carpenter KC, et al. International society of sports nutrition position stand: probiotics. J Int Soc Sports Nutr 2019;16:62.
85. Browne HP, Shao Y, Lawley TD. Mother-infant transmission of human microbiota. Curr Opin Microbiol 2022;69:102173.
86. Vatanen T, Franzosa EA, Schwager R, et al. The human gut microbiome in early-onset type 1 diabetes from the TEDDY study. Nature 2018;562:589-94.
87. Lawson MAE, O’Neill IJ, Kujawska M, et al. Breast milk-derived human milk oligosaccharides promote Bifidobacterium interactions within a single ecosystem. ISME J 2020;14:635-48.
88. Sakanaka M, Hansen ME, Gotoh A, et al. Evolutionary adaptation in fucosyllactose uptake systems supports bifidobacteria-infant symbiosis. Sci Adv 2019;5:eaaw7696.
89. Schöpping M, Gaspar P, Neves AR, Franzén CJ, Zeidan AA. Identifying the essential nutritional requirements of the probiotic bacteria Bifidobacterium animalis and Bifidobacterium longum through genome-scale modeling. NPJ Syst Biol Appl 2021;7:47.
90. Alessandri G, van Sinderen D, Ventura M. The genus Bifidobacterium: from genomics to functionality of an important component of the mammalian gut microbiota running title: Bifidobacterial adaptation to and interaction with the host. Comput Struct Biotechnol J 2021;19:1472-87.
91. Tarracchini C, Lugli GA, Mancabelli L, et al. Exploring the vitamin biosynthesis landscape of the human gut microbiota. mSystems 2024:e0092924.
92. Alessandri G, Ossiprandi MC, MacSharry J, van Sinderen D, Ventura M. Bifidobacterial dialogue with its human host and consequent modulation of the immune system. Front Immunol 2019;10:2348.
93. Rivière A, Selak M, Lantin D, Leroy F, De Vuyst L. Bifidobacteria and butyrate-producing colon bacteria: importance and strategies for their stimulation in the human gut. Front Microbiol 2016;7:979.
94. Duranti S, Ruiz L, Lugli GA, et al. Bifidobacterium adolescentis as a key member of the human gut microbiota in the production of GABA. Sci Rep 2020;10:14112.
95. Tojo R, Suárez A, Clemente MG, et al. Intestinal microbiota in health and disease: role of bifidobacteria in gut homeostasis. World J Gastroenterol 2014;20:15163-76.
96. Forootan M, Bagheri N, Darvishi M. Chronic constipation: a review of literature. Medicine 2018;97:e10631.
97. Wang L, Wang L, Tian P, et al. A randomised, double-blind, placebo-controlled trial of Bifidobacterium bifidum CCFM16 for manipulation of the gut microbiota and relief from chronic constipation. Food Funct 2022;13:1628-40.
98. Fuyuki A, Higurashi T, Kessoku T, et al. Efficacy of Bifidobacterium bifidum G9-1 in improving quality of life in patients with chronic constipation: a prospective intervention study. Biosci Microbiota Food Health 2021;40:105-14.
99. Makizaki Y, Uemoto T, Yokota H, Yamamoto M, Tanaka Y, Ohno H. Improvement of loperamide-induced slow transit constipation by Bifidobacterium bifidum G9-1 is mediated by the correction of butyrate production and neurotransmitter profile due to improvement in dysbiosis. PLoS One 2021;16:e0248584.
100. Nakamura Y, Suzuki S, Murakami S, et al. Integrated gut microbiome and metabolome analyses identified fecal biomarkers for bowel movement regulation by Bifidobacterium longum BB536 supplementation: A RCT. Comput Struct Biotechnol J 2022;20:5847-58.
101. Wang L, Hu L, Xu Q, et al. Bifidobacteria exert species-specific effects on constipation in BALB/c mice. Food Funct 2017;8:3587-600.
102. Wang L, Chai M, Wang J, et al. Bifidobacterium longum relieves constipation by regulating the intestinal barrier of mice. Food Funct 2022;13:5037-49.
103. Becattini S, Taur Y, Pamer EG. Antibiotic-induced changes in the intestinal microbiota and disease. Trends Mol Med 2016;22:458-78.
104. Patangia DV, Anthony Ryan C, Dempsey E, Paul Ross R, Stanton C. Impact of antibiotics on the human microbiome and consequences for host health. Microbiologyopen 2022;11:e1260.
105. Willing BP, Russell SL, Finlay BB. Shifting the balance: antibiotic effects on host-microbiota mutualism. Nat Rev Microbiol 2011;9:233-43.
106. Dahiya D, Nigam PS. Antibiotic-therapy-induced gut dysbiosis affecting gut microbiota-brain axis and cognition: restoration by intake of probiotics and synbiotics. Int J Mol Sci 2023;24:3074.
107. Dahiya D, Nigam PS. The gut microbiota influenced by the intake of probiotics and functional foods with prebiotics can sustain wellness and alleviate certain ailments like gut-inflammation and colon-cancer. Microorganisms 2022;10:665.
108. Duvallet C, Gibbons SM, Gurry T, Irizarry RA, Alm EJ. Meta-analysis of gut microbiome studies identifies disease-specific and shared responses. Nat Commun 2017;8:1784.
109. Wirbel J, Pyl PT, Kartal E, et al. Meta-analysis of fecal metagenomes reveals global microbial signatures that are specific for colorectal cancer. Nat Med 2019;25:679-89.
110. Feng C, Zhang W, Zhang T, et al. Heat-killed Bifidobacterium bifidum B1628 May alleviate dextran sulfate sodium-induced colitis in mice, and the anti-inflammatory effect is associated with gut microbiota modulation. Nutrients 2022;14:5233.
111. Makizaki Y, Maeda A, Oikawa Y, et al. Probiotic Bifidobacterium bifidum G9-1 ameliorates phytohemagglutinin-induced diarrhea caused by intestinal dysbiosis. Microbiol Immunol 2019;63:481-6.
112. Kawahara T, Makizaki Y, Oikawa Y, et al. Oral administration of Bifidobacterium bifidum G9-1 alleviates rotavirus gastroenteritis through regulation of intestinal homeostasis by inducing mucosal protective factors. PLoS One 2017;12:e0173979.
113. Okai S, Usui F, Ohta M, et al. Intestinal IgA as a modulator of the gut microbiota. Gut Microbes 2017;8:486-92.
114. Michels M, Córneo E, Cucker L, et al. Bifidobacterium lactis CCT 7858 improves gastrointestinal symptoms by antibiotics treatment: a double-blind, randomized, placebo-controlled trial. Probiotics Antimicrob Proteins 2023;15:738-48.
115. Merenstein D, Fraser CM, Roberts RF, et al. Bifidobacterium animalis subsp. lactis BB-12 protects against antibiotic-induced functional and compositional changes in human fecal microbiome. Nutrients 2021;13:2814.
116. Xu B, Liang S, Zhao J, et al. Bifidobacterium animalis subsp. lactis XLTG11 improves antibiotic-related diarrhea by alleviating inflammation, enhancing intestinal barrier function and regulating intestinal flora. Food Funct 2022;13:6404-18.
117. Guarino A, Aguilar J, Berkley J, et al. Acute gastroenteritis in children of the world: what needs to be done? J Pediatr Gastroenterol Nutr 2020;70:694-701.
118. Tate JE, Burton AH, Boschi-Pinto C, Steele AD, Duque J, Parashar UD; WHO-coordinated Global Rotavirus Surveillance Network. 2008 estimate of worldwide rotavirus-associated mortality in children younger than 5 years before the introduction of universal rotavirus vaccination programmes: a systematic review and meta-analysis. Lancet Infect Dis 2012;12:136-41.
119. Patel MM, Widdowson MA, Glass RI, Akazawa K, Vinjé J, Parashar UD. Systematic literature review of role of noroviruses in sporadic gastroenteritis. Emerg Infect Dis 2008;14:1224-31.
120. Rackoff LA, Bok K, Green KY, Kapikian AZ. Epidemiology and evolution of rotaviruses and noroviruses from an archival WHO Global Study in Children (1976-79) with implications for vaccine design. PLoS One 2013;8:e59394.
121. Walker CLF, Rudan I, Liu L, et al. Global burden of childhood pneumonia and diarrhoea. Lancet 2013;381:1405-16.
122. Lanata CF, Fischer-Walker CL, Olascoaga AC, Torres CX, Aryee MJ, Black RE; Child Health Epidemiology Reference Group of the World Health Organization and UNICEF. Global causes of diarrheal disease mortality in children <5 years of age: a systematic review. PLoS One 2013;8:e72788.
123. Nirwati H, Donato CM, Mawarti Y, et al. Norovirus and rotavirus infections in children less than five years of age hospitalized with acute gastroenteritis in Indonesia. Arch Virol 2019;164:1515-25.
124. Galán NN, Ulloa Rubiano JC, Velez Reyes FA, Fernandez Duarte KP, Salas Cárdenas SP, Gutierrez Fernandez MF. In vitro antiviral activity of Lactobacillus casei and Bifidobacterium adolescentis against rotavirus infection monitored by NSP4 protein production. J Appl Microbiol 2016;120:1041-51.
125. Gonzalez-Ochoa G, Flores-Mendoza LK, Icedo-Garcia R, Gomez-Flores R, Tamez-Guerra P. Modulation of rotavirus severe gastroenteritis by the combination of probiotics and prebiotics. Arch Microbiol 2017;199:953-61.
126. Li D, Breiman A, le Pendu J, Uyttendaele M. Anti-viral effect of Bifidobacterium adolescentis against noroviruses. Front Microbiol 2016;7:864.
127. Fernandez-Duarte KP, Olaya-Galán NN, Salas-Cárdenas SP, Lopez-Rozo J, Gutierrez-Fernandez MF. Bifidobacterium adolescentis (DSM 20083) and Lactobacillus casei (Lafti L26-DSL): probiotics able to block the in vitro adherence of rotavirus in MA104 cells. Probiotics Antimicrob Proteins 2018;10:56-63.
128. Wittmann A, Autenrieth IB, Frick JS. Plasmacytoid dendritic cells are crucial in Bifidobacterium adolescentis-mediated inhibition of Yersinia enterocolitica infection. PLoS One 2013;8:e71338.
129. Kim MJ, Lee DK, Park JE, Park IH, Seo JG, Ha NJ. Antiviral activity of Bifidobacterium adolescentis SPM1605 against Coxsackievirus B3. Biotechnol Biotechnol Equip 2014;28:681-8.
131. Culpepper T, Christman MC, Nieves C Jr, et al. Bifidobacterium bifidum R0071 decreases stress-associated diarrhoea-related symptoms and self-reported stress: a secondary analysis of a randomised trial. Benef Microbes 2016;7:327-36.
132. Koloski NA, Jones M, Kalantar J, Weltman M, Zaguirre J, Talley NJ. The brain--gut pathway in functional gastrointestinal disorders is bidirectional: a 12-year prospective population-based study. Gut 2012;61:1284-90.
133. Hughes C, Davoodi-Semiromi Y, Colee JC, et al. Galactooligosaccharide supplementation reduces stress-induced gastrointestinal dysfunction and days of cold or flu: a randomized, double-blind, controlled trial in healthy university students. Am J Clin Nutr 2011;93:1305-11.
134. Roos NM, Katan MB. Effects of probiotic bacteria on diarrhea, lipid metabolism, and carcinogenesis: a review of papers published between 1988 and 1998. Am J Clin Nutr 2000;71:405-11.
135. Gomi A, Harima-Mizusawa N, Shibahara-Sone H, Kano M, Miyazaki K, Ishikawa F. Effect of Bifidobacterium bifidum BF-1 on gastric protection and mucin production in an acute gastric injury rat model. J Dairy Sci 2013;96:832-7.
136. Urita Y, Goto M, Watanabe T, et al. Continuous consumption of fermented milk containing Bifidobacterium bifidum YIT 10347 improves gastrointestinal and psychological symptoms in patients with functional gastrointestinal disorders. Biosci Microbiota Food Health 2015;34:37-44.
137. Guandalini S, Sansotta N. Probiotics in the treatment of inflammatory bowel disease. In: Guandalini S, Indrio F, editors. Probiotics and child gastrointestinal health. Cham: Springer; 2019. pp. 101-7.
138. O’Callaghan A, van Sinderen D. Bifidobacteria and their role as members of the human gut microbiota. Front Microbiol 2016;7:925.
139. Bosselaar S, Dhelin L, Dautel E, et al. Taxonomic and phenotypic analysis of bifidobacteria isolated from IBD patients as potential probiotic strains. BMC Microbiol 2024;24:233.
140. Duranti S, Gaiani F, Mancabelli L, et al. Elucidating the gut microbiome of ulcerative colitis: bifidobacteria as novel microbial biomarkers. FEMS Microbiol Ecol 2016;92:fiw191.
141. Vavricka SR, Schoepfer A, Scharl M, Lakatos PL, Navarini A, Rogler G. Extraintestinal manifestations of inflammatory bowel disease. Inflamm Bowel Dis 2015;21:1982-92.
142. Peng C, Li J, Miao Z, et al. Early life administration of Bifidobacterium bifidum BD-1 alleviates long-term colitis by remodeling the gut microbiota and promoting intestinal barrier development. Front Microbiol 2022;13:916824.
143. Ishikawa H, Matsumoto S, Ohashi Y, et al. Beneficial effects of probiotic Bifidobacterium and galacto-oligosaccharide in patients with ulcerative colitis: a randomized controlled study. Digestion 2011;84:128-33.
144. Guglielmetti S, Mora D, Gschwender M, Popp K. Randomised clinical trial: Bifidobacterium bifidum MIMBb75 significantly alleviates irritable bowel syndrome and improves quality of life--a double-blind, placebo-controlled study. Aliment Pharmacol Ther 2011;33:1123-32.
145. Tomita T, Fukui H, Okugawa T, et al. Effect of Bifidobacterium bifidum G9-1 on the intestinal environment and diarrhea-predominant irritable bowel syndrome (IBS-D)-like symptoms in patients with quiescent Crohn’s disease: a prospective pilot study. J Clin Med 2023;12:3368.
146. Andresen V, Gschossmann J, Layer P. Heat-inactivated Bifidobacterium bifidum MIMBb75 (SYN-HI-001) in the treatment of irritable bowel syndrome: a multicentre, randomised, double-blind, placebo-controlled clinical trial. Lancet Gastroenterol Hepatol 2020;5:658-66.
147. Lenoir M, Wienke J, Fardao-Beyler F, Roese N. An 8-week course of Bifidobacterium longum 35624® is associated with a reduction in the symptoms of irritable bowel syndrome. Probiotics Antimicrob Proteins 2023;Online ahead of print.
148. Sabaté JM, Iglicki F. Effect of Bifidobacterium longum 35624 on disease severity and quality of life in patients with irritable bowel syndrome. World J Gastroenterol 2022;28:732-44.
149. He C, Xie Y, Zhu Y, et al. Probiotics modulate gastrointestinal microbiota after Helicobacter pylori eradication: A multicenter randomized double-blind placebo-controlled trial. Front Immunol 2022;13:1033063.
150. Gomi A, Yamaji K, Watanabe O, et al. Bifidobacterium bifidum YIT 10347 fermented milk exerts beneficial effects on gastrointestinal discomfort and symptoms in healthy adults: a double-blind, randomized, placebo-controlled study. J Dairy Sci 2018;101:4830-41.
151. Cheng J, Ouwehand AC. Gastroesophageal reflux disease and probiotics: a systematic review. Nutrients 2020;12:132.
152. Zheng Y, Zhang S, Zhang T, et al. A Bifidobacterium animalis subsp. lactis strain that can suppress Helicobacter pylori: isolation, in vitro and in vivo validation. Lett Appl Microbiol 2024;77:ovae005.
153. Marteau P, Le Nevé B, Quinquis L, Pichon C, Whorwell PJ, Guyonnet D. Consumption of a fermented milk product containing Bifidobacterium lactis CNCM I-2494 in women complaining of minor digestive symptoms: rapid response which is independent of dietary fibre intake or physical activity. Nutrients 2019;11:92.
154. Chibbar R, Dieleman LA. The gut microbiota in celiac disease and probiotics. Nutrients 2019;11:2375.
155. Pecora F, Persico F, Gismondi P, et al. Gut microbiota in celiac disease: is there any role for probiotics? Front Immunol 2020;11:957.
156. Klemenak M, Dolinšek J, Langerholc T, Di Gioia D, Mičetić-Turk D. Administration of Bifidobacterium breve decreases the production of TNF-α in children with celiac disease. Dig Dis Sci 2015;60:3386-92.
157. Quagliariello A, Aloisio I, Bozzi Cionci N, et al. Effect of Bifidobacterium breve on the intestinal microbiota of coeliac children on a gluten free diet: a pilot study. Nutrients 2016;8:660.
158. Olivares M, Laparra M, Sanz Y. Influence of Bifidobacterium longum CECT 7347 and gliadin peptides on intestinal epithelial cell proteome. J Agric Food Chem 2011;59:7666-71.
159. Medina M, De Palma G, Ribes-Koninckx C, Calabuig M, Sanz Y. Bifidobacterium strains suppress in vitro the pro-inflammatory milieu triggered by the large intestinal microbiota of coeliac patients. J Inflamm 2008;5:19.
160. Laparra JM, Olivares M, Gallina O, Sanz Y. Bifidobacterium longum CECT 7347 modulates immune responses in a gliadin-induced enteropathy animal model. PLoS One 2012;7:e30744.
161. Guardamagna O, Amaretti A, Puddu PE, et al. Bifidobacteria supplementation: effects on plasma lipid profiles in dyslipidemic children. Nutrition 2014;30:831-6.
162. Bordoni A, Amaretti A, Leonardi A, et al. Cholesterol-lowering probiotics: in vitro selection and in vivo testing of bifidobacteria. Appl Microbiol Biotechnol 2013;97:8273-81.
163. Zanotti I, Turroni F, Piemontese A, et al. Evidence for cholesterol-lowering activity by Bifidobacterium bifidum PRL2010 through gut microbiota modulation. Appl Microbiol Biotechnol 2015;99:6813-29.
164. Minami JI, Kondo S, Yanagisawa N, et al. Oral administration of Bifidobacterium breve B-3 modifies metabolic functions in adults with obese tendencies in a randomised controlled trial. J Nutr Sci 2015;4:e17.
165. Minami J, Iwabuchi N, Tanaka M, et al. Effects of Bifidobacterium breve B-3 on body fat reductions in pre-obese adults: a randomized, double-blind, placebo-controlled trial. Biosci Microbiota Food Health 2018;37:67-75.
166. Koutnikova H, Genser B, Monteiro-Sepulveda M, et al. Impact of bacterial probiotics on obesity, diabetes and non-alcoholic fatty liver disease related variables: a systematic review and meta-analysis of randomised controlled trials. BMJ Open 2019;9:e017995.
167. Ming J, Yu X, Xu X, et al. Effectiveness and safety of Bifidobacterium and berberine in human hyperglycemia and their regulatory effect on the gut microbiota: a multi-center, double-blind, randomized, parallel-controlled study. Genome Med 2021;13:125.
168. Qian X, Si Q, Lin G, et al. Bifidobacterium adolescentis is effective in relieving type 2 diabetes and may be related to its dominant core genome and gut microbiota modulation capacity. Nutrients 2022;14:2479.
169. Chen J, Wang R, Li XF, Wang RL. Bifidobacterium adolescentis supplementation ameliorates visceral fat accumulation and insulin sensitivity in an experimental model of the metabolic syndrome. Br J Nutr 2012;107:1429-34.
170. Wang B, Kong Q, Cui S, et al. Bifidobacterium adolescentis isolated from different hosts modifies the intestinal microbiota and displays differential metabolic and immunomodulatory properties in mice fed a high-fat diet. Nutrients 2021;13:1017.
171. Taipale TJ, Pienihäkkinen K, Isolauri E, Jokela JT, Söderling EM. Bifidobacterium animalis subsp. lactis BB-12 in reducing the risk of infections in early childhood. Pediatr Res 2016;79:65-9.
172. Taipale TJ, Pienihäkkinen K, Isolauri E, et al. Bifidobacterium animalis subsp. lactis BB-12 in reducing the risk of infections in infancy. Br J Nutr 2011;105:409-16.
173. Dekker J, Quilter M, Qian H. Comparison of two probiotics in follow-on formula: Bifidobacterium animalis subsp. lactis HN019 reduced upper respiratory tract infections in Chinese infants. Benef Microbes 2022;13:341-54.
174. Hevia A, Milani C, López P, et al. Allergic patients with long-term asthma display low levels of Bifidobacterium adolescentis. PLoS One 2016;11:e0147809.
175. Casaro MC, Crisma AR, Vieira AT, et al. Prophylactic Bifidobacterium adolescentis ATTCC 15703 supplementation reduces partially allergic airway disease in Balb/c but not in C57BL/6 mice. Benef Microbes 2018;9:465-76.
176. Fan Z, Yang B, Ross RP, et al. Protective effects of Bifidobacterium adolescentis on collagen-induced arthritis in rats depend on timing of administration. Food Funct 2020;11:4499-511.
177. Ferro M, Charneca S, Dourado E, Guerreiro CS, Fonseca JE. Probiotic supplementation for rheumatoid arthritis: a promising adjuvant therapy in the gut microbiome era. Front Pharmacol 2021;12:711788.
178. Lin L, Zhang K, Xiong Q, et al. Gut microbiota in pre-clinical rheumatoid arthritis: From pathogenesis to preventing progression. J Autoimmun 2023;141:103001.
179. Bodkhe R, Balakrishnan B, Taneja V. The role of microbiome in rheumatoid arthritis treatment. Ther Adv Musculoskelet Dis 2019;11:1759720X19844632.
180. Scher JU, Abramson SB. Periodontal disease, Porphyromonas gingivalis, and rheumatoid arthritis: what triggers autoimmunity and clinical disease? Arthritis Res Ther 2013;15:122.
181. Mikuls TR, Payne JB, Reinhardt RA, et al. Antibody responses to Porphyromonas gingivalis (P. gingivalis) in subjects with rheumatoid arthritis and periodontitis. Int Immunopharmacol 2009;9:38-42.
182. Hojo K, Nagaoka S, Murata S, Taketomo N, Ohshima T, Maeda N. Reduction of vitamin K concentration by salivary Bifidobacterium strains and their possible nutritional competition with Porphyromonas gingivalis. J Appl Microbiol 2007;103:1969-74.
183. Li B, Ding M, Liu X, et al. Bifidobacterium breve CCFM1078 alleviates collagen-induced arthritis in rats via modulating the gut microbiota and repairing the intestinal barrier damage. J Agric Food Chem 2022;70:14665-78.
184. Achi SC, Talahalli RR, Halami PM. Prophylactic effects of probiotic Bifidobacterium spp. in the resolution of inflammation in arthritic rats. Appl Microbiol Biotechnol 2019;103:6287-96.
186. Góralczyk-Bińkowska A, Szmajda-Krygier D, Kozłowska E. The microbiota-gut-brain axis in psychiatric disorders. Int J Mol Sci 2022;23:11245.
187. Gulas E, Wysiadecki G, Strzelecki D, Gawlik-Kotelnicka O, Polguj M. Can microbiology affect psychiatry? Psychiatr Pol 2018;52:1023-39.
188. Alli SR, Gorbovskaya I, Liu JCW, Kolla NJ, Brown L, Müller DJ. The gut microbiome in depression and potential benefit of prebiotics, probiotics and synbiotics: a systematic review of clinical trials and observational studies. Int J Mol Sci 2022;23:4494.
189. Sarasa SB, Mahendran R, Muthusamy G, Thankappan B, Selta DRF, Angayarkanni J. A brief review on the non-protein amino acid, gamma-amino butyric acid (GABA): its production and role in microbes. Curr Microbiol 2020;77:534-44.
190. Barrett E, Ross RP, O’Toole PW, Fitzgerald GF, Stanton C. γ-Aminobutyric acid production by culturable bacteria from the human intestine. J Appl Microbiol 2012;113:411-7.
192. Schousboe A, Bak LK, Sickmann HM, Sonnewald U, Waagepetersen HS. Energy substrates to support glutamatergic and GABAergic synaptic function: role of glycogen, glucose and lactate. Neurotox Res 2007;12:263-8.
193. Allen AP, Hutch W, Borre YE, et al. Bifidobacterium longum 1714 as a translational psychobiotic: modulation of stress, electrophysiology and neurocognition in healthy volunteers. Transl Psychiatry 2016;6:e939.
194. Kim CS, Cha L, Sim M, et al. Probiotic supplementation improves cognitive function and mood with changes in gut microbiota in community-dwelling older adults: a randomized, double-blind, placebo-controlled, multicenter trial. J Gerontol A Biol Sci Med Sci 2021;76:32-40.
195. Boehme M, Rémond-Derbez N, Lerond C, et al. Bifidobacterium longum subsp. longum reduces perceived psychological stress in healthy adults: an exploratory clinical trial. Nutrients 2023;15:3122.
196. Wang H, Braun C, Murphy EF, Enck P. Bifidobacterium longum 1714TM strain modulates brain activity of healthy volunteers during social stress. Am J Gastroenterol 2019;114:1152-62.
197. Bercik P, Park AJ, Sinclair D, et al. The anxiolytic effect of Bifidobacterium longum NCC3001 involves vagal pathways for gut-brain communication. Neurogastroenterol Motil 2011;23:1132-9.
198. Haas GS, Wang W, Saffar M, Mooney-Leber SM, Brummelte S. Probiotic treatment (Bifidobacterium longum subsp. longum 35624TM) affects stress responsivity in male rats after chronic corticosterone exposure. Behav Brain Res 2020;393:112718.
199. Tarracchini C, Viglioli M, Lugli GA, et al. The Integrated Probiotic Database: a genomic compendium of bifidobacterial health-promoting strains. Microbiome Res Rep 2022;1:9.
200. Turroni F, van Sinderen D, Ventura M. Bifidobacteria: insights into the biology of a key microbial group of early life gut microbiota. Microbiome Res Rep 2021;1:2.
201. Gerritsen J, Smidt H, Rijkers GT, de Vos WM. Intestinal microbiota in human health and disease: the impact of probiotics. Genes Nutr 2011;6:209-40.
Cite This Article

How to Cite
Bocchio, F.; Mancabelli L.; Milani C.; Lugli G. A.; Tarracchini C.; Longhi G.; Conto F. D.; Turroni F.; Ventura M. Compendium of Bifidobacterium-based probiotics: characteristics and therapeutic impact on human diseases. Microbiome. Res. Rep. 2024, 3, 51. http://dx.doi.org/10.20517/mrr.2024.52
Download Citation
Export Citation File:
Type of Import
Tips on Downloading Citation
Citation Manager File Format
Type of Import
Direct Import: When the Direct Import option is selected (the default state), a dialogue box will give you the option to Save or Open the downloaded citation data. Choosing Open will either launch your citation manager or give you a choice of applications with which to use the metadata. The Save option saves the file locally for later use.
Indirect Import: When the Indirect Import option is selected, the metadata is displayed and may be copied and pasted as needed.
About This Article
Copyright
Data & Comments
Data
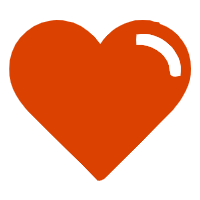
Comments
Comments must be written in English. Spam, offensive content, impersonation, and private information will not be permitted. If any comment is reported and identified as inappropriate content by OAE staff, the comment will be removed without notice. If you have any queries or need any help, please contact us at support@oaepublish.com.