Detection of urine circulating tumor DNA using droplet digital PCR for predicting hepatocellular carcinoma recurrence
Abstract
Aim: This study aims to evaluate the feasibility of examining circulating tumor DNA (ctDNA) in urine samples from hepatocellular carcinoma (HCC) patients by droplet digital PCR (ddPCR) and to assess its value in predicting HCC recurrence after surgery.
Methods: HCC cases who accepted surgical resection were included. Perioperative urine, tissue and peripheral blood specimens were collected. Four hotspot mutants [TP53-rs28934571 (c.747G>T), TRET-rs1242535815
Results: Forty-six patients were enrolled, and 18 patients (39.1%, 18/46) exhibited detectable circulating mutants, with the MAF in the range of 0.07% to 0.91%. The consistency test indicated moderate to substantial concordance between urine and paired tumor tissue mutations. The mutation level dropped dramatically or disappeared after surgery. Positive urine ctDNA before surgery was closely related to greater tumor size and recurrence. Kaplan-Meier curves revealed significantly shorter disease-free survival (DFS) for ctDNA-positive patients. Multivariate analysis identified detectable urine ctDNA as an independent risk factor for tumor recurrence. More than that, receiver operating characteristic (ROC) curves demonstrated that urine ctDNA had the largest area under the curve (AUC) for predicting HCC recurrence.
Conclusion: Detecting ctDNA in urine using ddPCR is feasible and holds significant potential for predicting and monitoring HCC recurrence.
Keywords
INTRODUCTION
Hepatocellular carcinoma (HCC) is one of the most common cancers and represents a major global health problem[1]. Although surgical resection remains the most effective treatment, many cases are diagnosed at an advanced stage, leading to poor outcomes. Additionally, postoperative recurrence significantly affects prognosis. Therefore, early screening, detection, and diagnosis are critical for improving outcomes. Currently, early detection and monitoring of HCC recurrence rely on imaging, tissue biopsy, and serum biomarkers[2,3]. However, imaging has limitations in diagnostic accuracy and sensitivity[4]. Tissue biopsy is invasive and inadequate for monitoring dynamic tumor progression[5]. Alpha-fetoprotein (AFP) is the most widely used serum biomarker for HCC screening and diagnosis, but nearly 30% of early HCC cases can be AFP-negative due to transcriptional regulation factors[6]. Even with a low-level cutoff, the specificity and sensitivity of AFP for HCC diagnosis remain inadequate[6,7]. Other biomarkers, such as AFP-L3, PIVKA-II, GPC-3, and microRNAs, demonstrate diagnostic value in cases where AFP is negative but still serve as alternative options[6,8]. Therefore, it is crucial to identify a novel and less invasive or non-invasive diagnostic strategy for early detection of HCC and monitoring its recurrence.
Over the past decades, the concept of “liquid biopsy” has gained significant attention[9]. Liquid biopsy involves the collection of body fluid samples (such as blood, urine, saliva, etc.) for subsequent testing. Circulating tumor DNA (ctDNA) serves as the foundation of liquid biopsy[10]. It is widely acknowledged that ctDNAs are mutated DNA fragments released into the bloodstream by primary or metastatic tumors, constituting a portion of circulating cell-free DNA (cfDNA)[11]. ctDNA carries genetic abnormalities identical to those found in the originating tumor cells, enabling researchers to distinguish it from cfDNA and develop effective detection methods[12,13].
Prior studies have demonstrated the feasibility of detecting ctDNA from peripheral blood to profile tumor genomes, guide therapy, monitor tumor progression, and unveil drug resistance in various cancers, including HCC[14,15]. Furthermore, other researchers have determined urinary specimens to be potentially useful for ctDNA extraction in several diseases[9,16-18]. Compared to blood and tissue collection, urinary samples are non-invasive and easily obtainable, making them more convenient for disease monitoring. Therefore, patients are more likely to accept this method in clinical practice.
In this study, our objective is to further assess the viability of utilizing urine samples as an alternative to tissue and blood plasma for the identification and characterization of specific mutations in four previously documented DNA modifications associated with HCC[15]. We evaluated the feasibility of detecting ctDNA through the utilization of droplet digital PCR (ddPCR) targeting hotspot mutations within urine samples. Subsequently, we compared the presence of mutants detected in urine with their corresponding status in paired tumor tissue samples, while also evaluating the diagnostic efficacy and predictive value for recurrence.
METHODS
Patients and samples
Forty-six tumor tissues and urine samples were collected from patients hospitalized for curative resection at Zhongshan Hospital, Fudan University, between March and November 2018. The eligibility criteria included: (1) pathologically proven HCC; (2) no previous history of any malignancies; (3) absence of synchronous malignancies in other organs; (4) no prior use of any form of antitumor therapies. Tissue samples were collected immediately after resection and stored at -80 °C until use. At least 50 mL of midstream urine was collected in the morning at indicated time points and processed within 2 h. To remove debris and cellular contaminants, urine specimens were spun at 15,000 g for 15 min and at least 5 mL of supernatant was recovered. Subsequent preparations for urine cfDNA extraction were described in a previous study[18].
Urine cfDNA extraction and ddPCR for the detection of ctDNA
Urine cfDNA was purified and extracted using the QIAamp Circulating Nucleic Acid Kit (QIAGEN, Inc, USA) with the QIAvac 24 Plus vacuum manifold, following the manufacturer’s instructions. The ddPCR was conducted on the QX200 ddPCR platform (Bio-Rad, Hercules, USA). The ddPCR reaction system consisted of 20 μL volumes, including 10 μL 2× ddPCR SuperMix (Bio-Rad), 5 μL cfDNA template, 1 μL (20×) wild type probe/primer mix, 1 μL
Sanger sequencing
The DNeasy Blood & Tissue Kit (QIAGEN, Inc, USA) was used for DNA extraction from tumors and matched adjacent liver tissues. PCR products were purified using the MinElute PCR Purification Kit (QIAGEN). Detailed procedures are available in a previous report[14].
Patient follow-ups
The patients were scheduled for follow-up appointments at 1 month post-surgery, then every 3 months during the first two years, and subsequently every 6 months. The follow-up assessments consisted of abdominal ultrasonography, monitoring serum tumor biomarker levels, and conducting enhanced abdominal computed tomography (CT) or magnetic resonance imaging (MRI) if deemed necessary. Patient monitoring continued until the recurrence of the disease, death, or completion of the study period. Disease-free survival (DFS) denoted the duration between the surgical procedure and disease recurrence.
Statistical analysis
Statistical analysis was conducted using SPSS 27.0 (IBM, Chicago, IL, USA) and GraphPad Prism 9 for Mac (GraphPad Software, San Diego, California, USA). Student t-tests were employed for comparison between groups. The chi-square test was utilized to compare clinical parameters between groups. The Kaplan-Meier method was used to calculate cumulative survival time and the log-rank test was applied for analysis. Univariate and multivariate analyses were performed based on the Cox proportional hazards regression model. For experiments carried out in duplicate, data were presented as mean ± standard error. All statistical tests were two-tailed with a significance level set at P < 0.05.
RESULTS
Patients’ demographics
We included a total of 46 pathologically diagnosed HCC patients in this study. Patients’ clinicopathological and epidemiological information are summarized in Table 1. The majority of the patients were male (37/46, 80.4%) with a median age of 59 years (range 35-82). Among them, 37 patients tested positive for hepatitis B surface antigen (HbsAg) and 39 were diagnosed with liver cirrhosis. Prior to the surgical procedure, AFP levels above 20 ng/mL were observed in 27 patients, while a Child-Pugh score of A was recorded in 44 patients. Referring to the most recent guidelines on primary liver cancer in China[4], the clinical stages of the patients were distributed as follows: Ia (15 cases), Ib (25 cases), IIa (4 cases), IIb (2 cases). All patients underwent successful hepatectomy with curative intent.
Clinical characteristics of enrolled patients
Clinical characteristics | Number of patients |
Age, years | |
≥ 60 | 22 (median 59) |
< 60 | 24 |
Gender | |
Male | 37 |
Female | 9 |
HBsAg | |
Negative | 9 |
Positive | 37 |
Cirrhosis | |
No | 7 |
Yes | 39 |
Tumor size | |
≥ 5 cm | 22 |
< 5 cm | 24 |
Tumor number | |
Single | 34 |
Multiple | 12 |
MVI | |
No | 23 |
Yes | 23 |
Tumor encapsulation | |
No | 16 |
Yes | 30 |
Edmonson grade | |
I + II | 33 |
III + IV | 13 |
AFP | |
< 20 ng/mL | 19 |
≥ 20 ng/mL | 27 |
ALT | |
< 50 μ/L | 41 |
≥ 50 μ/L | 5 |
AST | |
< 40 μ/L | 13 |
≥ 40 μ/L | 33 |
Child-Pugh score | |
A | 44 |
B | 2 |
Detection of circulating mutant DNA in the urine of HCC patients using ddPCR
The QX200 reaction platform’s ability to detect low levels of analytes (LOD) and maintain working stability has been previously assessed in our earlier studies[14,15]. In these investigations, we established the LOD for the ddPCR platform at 0.01% with excellent reproducibility. To guide subsequent experiments, we identified 4 frequently reported mutation sites within 3 genes (TP53 c.747G>T, TERT c.1-124C>T, CTNNB1 c.121A>G and c.133T>C) in HCC, based on findings from previous whole genome/exome sequencing research and data from the COSMIC database.
To begin with, we conducted an assessment on the possibility of identifying these 4 mutations in cfDNA obtained from urine using ddPCR. We successfully extracted cfDNA from urine samples with concentrations ranging from 18-30 ng/μL, which met the detection requirements. A total of 18 out of 46 patients (39.1%) were identified to have at least one mutation in urine before surgery. Among them, 7 patients had TP53 (c.747G>T) positive; 9 patients were positive for TERT (c.1-124C>T); 6 patients were found to have CTNNB1 (c.121A>G) mutants; and 5 patients had detectable CTNNB1 (c.133T>C) mutants [Table 2]. Generally speaking, the MAFs ranged from 0.07% to 0.91%, all surpassing the LOD level of the ddPCR platform for detection purposes. Multiple mutations were simultaneously detected in 6 patients.
Analysis of mutation status in urine and plasma with ddPCR
Patient | Mutation fraction (mutant/wild type, copies/μL) | |||
TP53 (c.747G>T) | TERT (c.1-124C>T) | CTNB1 (c.121A>G) | CTNB1 (c.133T>C) | |
urine/tissue | urine/tissue | urine/tissue | urine/tissue | |
H02 | 0.2%/0.34% | 0.11%/NA | 0.08%/NA | 0.07%/0.04% |
H03 | NA | 0.21%/NA | ||
H08 | NA | 0.13%/1.02% | ||
H10 | NA/0.18% | |||
H11 | NA/0.12% | |||
H12 | NA/0.12% | |||
H13 | 0.25%/3.2% | 0.27%/2.19% | ||
H15 | 0.31%/1.2% | |||
H16 | 0.14%/NA | 0.1%/NA | 0.22%/0.63% | |
H19 | 0.7%/NA | 0.72%/NA | ||
H20 | 0.29%/0.93% | |||
H23 | 0.18%/0.59% | |||
H25 | NA/2.19% | |||
H30 | 0.23%/NA | |||
H32 | 0.66%/3.11% | |||
H34 | 0.34%/1.39% | 0.19%/NA | ||
H37 | 0.21%/4.3% | NA/0.04% | ||
H40 | 0.91%/3.63% | |||
H41 | 0.58%/2.77% | |||
H42 | 0.67%/5.22% | |||
H43 | 0.81%/3.6% | 0.16%/0.63% | ||
H44 | 0.67%/4.34% |
Utilize sanger sequencing to determine the source of urine mutants by analyzing paired tumor tissues and peripheral blood mononuclear cells
We conducted DNA sequencing of tumor tissues and corresponding liver tissues or peripheral blood mononuclear cells (PBMCs) in order to eliminate the possibility of detecting germline mutations that may also be presented in urine cfDNA. Sanger sequencing was carried out on all samples, irrespective of the mutant status observed in cfDNA. Negative results of Sanger sequencing could also be found in our previously published article[14]. In the cases of five patients whose cfDNAs showed no mutations, tumor tissues revealed a heterozygous genotype of TP53 (c.747G>T) and CTNNB1. Conversely, in six patients (H02, H03, H16, H19, H30, and H34) with circulating mutants, their corresponding tumor tissues tested negative [Table 2]. No mutations were detected in DNAs extracted from adjacent liver tissues or PBMCs, ruling out the possibility of germline mutations. A consistency test using the Kappa value demonstrated moderate to substantial agreement between urine mutants identified by ddPCR and their status in paired tumor tissues [Table 3].
Concordance of mutants detected in urine by ddPCR and their status in paired tumor tissues
Urine DNA | Tumor tissue | Total | Kappa value (95%CI) | P value | |
+ | - | ||||
TP53 (747G>T) | |||||
+ | 4 | 3 | 7 | 0.443 (0.036-0.761) | 0.003 |
- | 4 | 35 | 39 | ||
Total | 8 | 38 | 46 | ||
TERT (c.1-124C>T) | |||||
+ | 5 | 4 | 9 | 0.668 (0.315-0.920) | 0.001 |
- | 0 | 37 | 37 | ||
Total | 5 | 41 | 46 | ||
CTNNB1 (c.121A>G) | |||||
+ | 4 | 2 | 6 | 0.777 (0.315-1.000) | 0.001 |
- | 0 | 40 | 40 | ||
Total | 4 | 42 | 46 | ||
CTNNB1(c.133T>C) | |||||
+ | 4 | 1 | 5 | 0.691 (0.179-1.000) | 0.001 |
- | 2 | 39 | 41 | ||
Total | 6 | 40 | 46 |
ctDNA dynamic changes after surgery and preoperative ctDNA status correlates with tumor recurrence
We also collected urine samples on the third day after operation to evaluate the status of ctDNA upon tumor resection. All four mutations were assessed regardless of their status before surgery. Results showed that mutation levels that were high before surgery dropped dramatically or disappeared. Mutant TP53 (c.747G>T) copies per 100,000 GEQ dropped from 532.0 ± 99.4 to 19.1 ± 14.1 (P: 0.0003). Mutant CTNNB1 (c.133T>C) copies per 100,000 GEQ dropped from 242.6 ± 45.9 to 0.8 ± 0.8 (P: 0.0008). Copies per 100,000 GEQ of CTNNB1 (c.121A>G) dropped from 354.7 ± 84.9 to 16.8 ± 16.01 (P: 0.0029). For TERT (c.1-124C>T), copies per 100,000 GEQ dropped from 483.4 ± 89.4 to 27.8 ± 18.8 (P: 0.0001) [Figure 1A-D].
Figure 1. Quantification of gene mutation levels in the urine of patients with HCC before and after operation. The number of mutant copies detected was normalized to a constant number of calculated GEQ of wild-type DNA across all samples to yield a concentration of mutant fragments. (A) Mutant TP53 (c.747G>T); (B) CTNNB1 (c.133T>C); (C) CTNNB1 (c.121A>G); and (D) TERT (c.1-124C>T) copies per 100,000 GEQ dropped dramatically after surgery. HCC: Hepatocellular carcinoma; GEQ: genome equivalents.
Subsequently, we conducted an examination of the association between preoperative ctDNA status and clinicopathological features. The presence of urine ctDNA prior to surgery was found to be significantly associated with increased tumor size (P: 0.03) and a higher incidence of tumor recurrence (P: 0.04) [Table 4].
Comparison of the clinical characteristics between preoperative ctDNA positive and negative group
Clinicopathologic characteristics | Negative group | Positive group | P value | ||
N | % | N | % | ||
Age, years | |||||
≤ 60 | 16 | 34.8 | 10 | 21.7 | 0.92 |
> 60 | 12 | 26.1 | 8 | 17.4 | |
Gender | |||||
Male | 23 | 50.0 | 14 | 30.4 | 0.72 |
Female | 5 | 10.9 | 4 | 8.7 | |
HBsAg | |||||
Negative | 5 | 10.9 | 4 | 8.7 | 0.72 |
Positive | 23 | 50.0 | 14 | 30.4 | |
HCV | |||||
Negative | 28 | 60.9 | 16 | 34.8 | 0.15 |
Positive | 0 | 0 | 2 | 4.3 | |
Tumor size | |||||
≥ 5 cm | 11 | 23.9 | 12 | 26.1 | 0.03 |
< 5 cm | 17 | 37.0 | 6 | 13.0 | |
Tumor number | |||||
Single | 18 | 39.1 | 16 | 34.8 | 0.06 |
Multiple | 10 | 21.7 | 2 | 4.3 | |
MVI | |||||
No | 15 | 32.6 | 8 | 17.4 | 0.55 |
Yes | 13 | 28.3 | 10 | 21.7 | |
Tumor encapsulation | |||||
No | 8 | 17.4 | 8 | 17.4 | 0.27 |
Yes | 20 | 43.5 | 10 | 21.7 | |
Edmonson grade | |||||
I + II | 15 | 32.6 | 6 | 13.2 | 0.18 |
III + IV | 13 | 28.3 | 12 | 26.1 | |
AFP | |||||
< 20 ng/mL | 11 | 23.9 | 8 | 17.4 | 0.73 |
≥ 20 ng/mL | 17 | 41.3 | 10 | 17.4 | |
ALT | |||||
< 75 μ/L | 27 | 58.7 | 16 | 34.8 | 0.55 |
≥ 75 μ/L | 1 | 2.2 | 2 | 4.3 | |
GGT | |||||
< 54 μ/L | 13 | 28.3 | 8 | 17.4 | 0.89 |
≥ 54 μ/L | 15 | 32.6 | 10 | 21.7 | |
Tumor recurrence | |||||
No | 14 | 30.4 | 4 | 8.8 | 0.04 |
Yes | 14 | 30.4 | 14 | 30.4 |
Using the Kaplan-Meier curve, a comparison was conducted on the DFS between two groups: those with positive ctDNA and those with negative ctDNA. The findings revealed that individuals with a positive ctDNA status prior to surgery exhibited a significantly shorter DFS (median DFS 15.0 months vs. 77.0 months; P: 0.003; HR: 3.79; 95%CI: 1.58-9.13) [Figure 2A]. Next, we used a Cox regression model to conduct univariate and multivariate analyses to identify risk factors for tumor recurrence from listed clinicopathological parameters. In univariate analysis, des-γ-carboxy prothrombin (DCP) and ctDNA status were identified as risk factors for tumor recurrence. Relevant multivariate analyses demonstrated that the status of ctDNA served as an independent risk factor for recurrence [Table 5]. The receiver operating characteristic (ROC) curves presented the greatest area under the curve (AUC) (0.75; P: 0.004; 95%CI: 0.61-0.89) of ctDNA MAF for predicting postoperative recurrence [Figure 2B].
Figure 2. Comparison of DFS between different groups and diagnostic ability for recurrence of various clinical indices. (A) Median DFS between preoperative ctDNA positive and negative patients was 15 months vs. 77 months, P: 0.003, HR: 2.88 (95%CI: 1.23-6.71); (B) Preoperative ctDNA status ranked first place in predicting postoperative recurrence among other parameters assessed with an AUC of 0.75. DFS: Disease-free survival; ctDNA: circulating tumor DNA; HR: hazard ratio; AUC: area under the curve; ROC: receiver operating characteristic; DCP: des-γ-carboxy prothrombin.
Univariate and multivariate analysis of preoperative indexes for recurrence by Cox regression model
Clinicopathologic parameters | Univariate | Multivariate | ||
HR (95%CI) | P value | HR (95%CI) | P value | |
Age (year): < 60 vs. ≥ 60 | 0.77 (0.36-1.66) | 0.50 | ||
Gender: female vs. male | 0.37 (0.11-1.26) | 0.11 | ||
AFP (ng/mL): < 20 vs. ≥ 20 | 0.76 (0.35-1.62) | 0.48 | ||
ALT (u/L): < 75 vs. ≥ 75 | 0.97 (0.13-7.62) | 0.98 | ||
GGT (u/L): < 54 vs. ≥ 54 | 1.33 (0.62-2.86) | 0.47 | ||
HBsAg: negative vs. positive | 0.65 (0.22-1.89) | 0.43 | ||
Tumor size (cm): ≤ 5 vs. > 5 | 1.86 (0.87-4.00) | 0.11 | ||
Tumor number: single vs. multiple | 1.13 (0.50-2.58) | 0.77 | ||
Tumor encapsulation: complete vs. none | 0.60 (0.27-1.37) | 0.23 | ||
Tumor differentiation stage: III-IV vs. I-II | 1.29 (0.60-2.77) | 0.52 | ||
Vascular invasion: no vs. yes | 0.68 (0.31-1.46) | 0.32 | ||
DCP (mAU/mL): < 40 vs. ≥ 40 | 2.43 (1.12-5.26) | 0.02 | 2.25 (1.03-4.92) | 0.04 |
ctDNA status: positive vs. negative | 2.80 (1.27-6.18) | 0.01 | 2.60 (1.16-5.80) | 0.02 |
DISCUSSION
Liquid biopsy is increasingly used for various clinical and investigational applications, particularly for early detection and dynamic monitoring of tumors[10]. Profiling cfDNA, a central part of liquid biopsy, has been shown to closely match the corresponding tumors[11,19]. With advancements in genetic sequencing technology, probing the molecular landscape of solid tumors via a simple non-invasive urine draw, rather than blood samples, has significant implications for tumor monitoring and recurrence surveillance[5]. Analyzing urine cfDNA has several advantages. Firstly, sample acquisition is entirely non-invasive, eliminating the need for venesection or a healthcare professional to be present during sampling[20,21]. Secondly, there are no restrictions on sampling location due to the ease of collection. Thirdly, urine samples may yield a higher ctDNA-to-cfDNA ratio than plasma[22]. Previous studies have demonstrated that cfDNAs in urine contain DNA derived from solid tumors, including HCC and other cancers[9,17,18,22]. These cfDNAs contain broad genetic information from the derived tumors, also known as ctDNA, functioning as a competitive tool for analyzing the tumor genome.
In this limited longitudinal study involving 46 cases, we successfully demonstrated the ability to detect ctDNA in urine samples obtained from patients with HCC (18 out of 46, representing a detection rate of 39.1%). Given that ctDNA typically constitutes only a small portion of total cfDNA, we employed ddPCR as an exceptionally sensitive detection technique. This method can detect mutations as low as 0.001%, overcoming the low fraction of ctDNA in urine cfDNA[23,24]. However, ddPCR can only target a limited number of mutations per reaction[25], and the throughput is also limited. Therefore, we preferred hotspot mutations and pre-selected targeted mutants in this study. As previously reported, four sites from TP53, CTNNB1, and TERT were selected as candidate targets, as they are among the most frequently mutated genes with multiple loci and mutation types according to the COSMIC database. We believe that adding more relevant hotspots could further improve the detection rate.
To rule out the presence of potential hereditary mutations identified in urine, we performed Sanger sequencing on tumor tissues to determine their source. Interestingly, certain circulating mutants were not detected in the tumor tissues. Consistent with expectations, these circulating mutants were also absent in adjacent liver tissues and PBMCs. Given the high sensitivity of the ddPCR platform, the absence of mutants in germline DNA, and no history of previous or simultaneous malignancy, we classified these positive signals observed in cfDNA as ctDNA originating from tumor cells. Another underlying reason for the discordance between urine and tumor tissue mutation status could be intratumor heterogeneity (ITH). ITH refers to the presence of different subpopulations of cancer cells within a tumor that exhibit distinct genetic, epigenetic, and transcriptomic profiles[26,27]. One advantage of liquid biopsy is its ability to capture molecular heterogeneity from distinct tumor lesions or components harboring different genetic alterations[28].
To accommodate the natural variation in urine samples, the reported copies are normalized to 100,000 wild-type GEQ. This results in a revised and more presentable minimum detectable threshold for specific mutants. As expected, the amount of ctDNA MAF dropped dramatically after the surgical removal of the tumor. Patients with ctDNA MAF before surgery had a higher risk of tumor recurrence. Both the ROC and DFS assessments indicated that urine ctDNA MAF could be a potential predictive factor for HCC recurrence, with diagnostic ability superior to AFP, DCP, and plasma ctDNA MAF. Surprisingly, the quality of urine ctDNA was comparable to plasma ctDNA, albeit at a much lower concentration. AUC comparisons with urine and plasma ctDNA showed differences in sensitivity and specificity. We assumed that processing a larger sample size may compensate for the gap.
Moreover, the presence of detectable ctDNA prior to surgical intervention was found to be associated with the size of the tumor and potentially with the number of tumors. Given that ctDNA is acknowledged as fragments of tumor DNA circulating in the bloodstream, it is plausible that larger tumor sizes and multiple tumor lesions could lead to an increased likelihood of ctDNA release. Interestingly, we observed instances where there was a rise or emergence of mutations following surgery, which may also be attributed to ITH. Another reason could be that touching and squeezing the tumor during surgery might stimulate ctDNA release. Moreover, the presence of minimally residual disease (MRD) is another factor that cannot be ignored. MRD refers to the small number of cancer cells that may remain in a patient’s body after treatment, which is undetectable using conventional methods[29]. Highly sensitive liquid biopsy assays have the advantage of detecting and characterizing MRD, reflecting the presence of tumor cells disseminated from the primary lesion to distant organs in patients without clinical or radiological signs of metastasis or residual tumor cells left behind after therapy, eventually leading to local recurrence[29].
Some limitations should be recognized here. This is a single-center retrospective study with a small sample size. We assumed that mutations harbored in ctDNA might link with more tumor characteristics with data from a larger sample size. While we did find associations between preoperative ctDNA status and tumor recurrence, it is crucial to give greater consideration to instances where ctDNA status turns positive following surgery. As increasing MAFs might indicate MRD, these cases are more likely to experience recurrence or metastasis. By dynamically monitoring urine ctDNA, these cases could be closely observed for early detection of tumor recurrence. This is what we should focus on in subsequent studies. Another interesting phenomenon was the presence of multiple ctDNA MAFs in some cases. These cases should be further evaluated to understand the association between tumor behavior and ITH. As the number of mutation sites available is limited, it becomes crucial to utilize a more comprehensive and well-established mutant panel for the analysis of ctDNA in HCC. This may entail employing multiplex ddPCR and targeted deep sequencing techniques on ctDNA samples, with a focus on frequently mutated genes associated with HCC[30]. Notably, the levels of urine ctDNA can fluctuate due to various reasons, such as hydration. Further studies are needed to identify a proper internal control for appropriately setting cutoffs for urine marker values and conducting dynamic monitoring[9].
In summary, we have successfully shown the possibility of utilizing ddPCR to identify urine ctDNA in patients with HCC. Urine ctDNA testing may have significant potential for predicting and monitoring HCC recurrence. A larger longitudinal study is being designed to track the dynamic changes of urine ctDNA after surgery to monitor HCC recurrence and to determine whether this method can overcome the limitations of traditional methods such as imaging evaluation and serum tumor biomarkers so as to provide an ideal tool for monitoring HCC recurrence.
DECLARATIONS
Authors’ contributions
Designed research: Zhang X, Huang PX, Fu XT
Performed research: Zhang X, Huang PX
Carried out data analysis: Zhang X, Ma B, Guo DZ, Huang A
Supervised research: Liu WR, Ding ZB, Shi YH, Fan J, Zhou J, Fu XT
Wrote the paper: Zhang X, Huang PX
All authors contributed to the conception and design of the study.
All authors reviewed the final manuscript.
Availability of data and materials
The datasets used and/or analyzed during the current study are available from the corresponding author upon reasonable request.
Financial support and sponsorship
This study was jointly supported by National Natural Science Foundation of China (82150004), Shanghai Municipal Health Commission (20224Y0285, R2022-010), Shanghai “Rising Stars of Medical Talent” Youth Development Program (Outstanding Youth Medical Talents), the Projects from the Shanghai Science and Technology Commission (19441905000 and 21140900300), Natural Science Funds of Shanghai (21ZR1413800, 20ZR1473100), the Projects from Science Foundation of Zhongshan Hospital, Fudan University (2021ZSCX28, 2020ZSLC31), the Shanghai Municipal Science and Technology Major Project, and the Shanghai Municipal Key Clinical Specialty.
Conflicts of interest
All authors declared that there are no conflicts of interest.
Ethics approval and consent to participate
This study was approved by the Ethics Committees of the leading center of Zhongshan Hospital of Fudan University and all participating centers (Approval No. B2020-299R). Written informed consent was obtained from patients at the participating institutions for the archival of their biospecimens and their use in future studies.
Consent for publication
Not applicable.
Copyright
© The Author(s) 2024.
REFERENCES
1. Vogel A, Meyer T, Sapisochin G, Salem R, Saborowski A. Hepatocellular carcinoma. Lancet 2022;400:1345-62.
2. Ye Q, Ling S, Zheng S, Xu X. Liquid biopsy in hepatocellular carcinoma: circulating tumor cells and circulating tumor DNA. Mol Cancer 2019;18:114.
3. Colombo F, Baldan F, Mazzucchelli S, et al. Evidence of distinct tumour-propagating cell populations with different properties in primary human hepatocellular carcinoma. PLoS One 2011;6:e21369.
4. Zhou J, Sun H, Wang Z, et al. Guidelines for the diagnosis and treatment of primary liver cancer (2022 Edition). Liver Cancer 2023;12:405-44.
5. Siravegna G, Marsoni S, Siena S, Bardelli A. Integrating liquid biopsies into the management of cancer. Nat Rev Clin Oncol 2017;14:531-48.
6. Luo P, Wu S, Yu Y, et al. Current status and perspective biomarkers in AFP negative HCC: towards screening for and diagnosing hepatocellular carcinoma at an earlier stage. Pathol Oncol Res 2020;26:599-603.
7. Trevisani F, D'Intino PE, Morselli-Labate AM, et al. Serum alpha-fetoprotein for diagnosis of hepatocellular carcinoma in patients with chronic liver disease: influence of HBsAg and anti-HCV status. J Hepatol 2001;34:570-5.
8. Cui R, He J, Zhang F, et al. Diagnostic value of protein induced by vitamin K absence (PIVKAII) and hepatoma-specific band of serum gamma-glutamyl transferase (GGTII) as hepatocellular carcinoma markers complementary to alpha-fetoprotein. Br J Cancer 2003;88:1878-82.
9. Hann HW, Jain S, Park G, Steffen JD, Song W, Su YH. Detection of urine DNA markers for monitoring recurrent hepatocellular carcinoma. Hepatoma Res 2017;3:105-11.
10. Diaz LA Jr, Bardelli A. Liquid biopsies: genotyping circulating tumor DNA. J Clin Oncol 2014;32:579-86.
11. Bettegowda C, Sausen M, Leary RJ, et al. Detection of circulating tumor DNA in early- and late-stage human malignancies. Sci Transl Med 2014;6:224ra24.
12. Chan KC, Hung EC, Woo JK, et al. Early detection of nasopharyngeal carcinoma by plasma Epstein-Barr virus DNA analysis in a surveillance program. Cancer 2013;119:1838-44.
13. Campitelli M, Jeannot E, Peter M, et al. Human papillomavirus mutational insertion: specific marker of circulating tumor DNA in cervical cancer patients. PLoS One 2012;7:e43393.
14. Huang A, Zhang X, Zhou SL, et al. Detecting circulating tumor DNA in hepatocellular carcinoma patients using droplet digital PCR is feasible and reflects intratumoral heterogeneity. J Cancer 2016;7:1907-14.
15. Wang J, Huang A, Wang YP, et al. Circulating tumor DNA correlates with microvascular invasion and predicts tumor recurrence of hepatocellular carcinoma. Ann Transl Med 2020;8:237.
16. Abdullaev SA, Minkabirova GM, Bezlepkin VG, Gaziev AI. Cell-free DNA in the urine of rats exposed to ionizing radiation. Radiat Environ Biophys 2015;54:297-304.
17. Chen S, Zhao J, Cui L, Liu Y. Urinary circulating DNA detection for dynamic tracking of EGFR mutations for NSCLC patients treated with EGFR-TKIs. Clin Transl Oncol 2017;19:332-40.
18. Husain H, Melnikova VO, Kosco K, et al. Monitoring daily dynamics of early tumor response to targeted therapy by detecting circulating tumor DNA in urine. Clin Cancer Res 2017;23:4716-23.
19. Karachaliou N, Mayo-de las Casas C, Queralt C, et al; Spanish Lung Cancer Group. Association of EGFR L858R mutation in circulating free DNA with survival in the EURTAC trial. JAMA Oncol 2015;1:149-57.
20. Tivey A, Church M, Rothwell D, Dive C, Cook N. Circulating tumour DNA - looking beyond the blood. Nat Rev Clin Oncol 2022;19:600-12.
21. Augustus E, Van Casteren K, Sorber L, et al. The art of obtaining a high yield of cell-free DNA from urine. PLoS One 2020;15:e0231058.
22. Green EA, Li R, Albiges L, et al. Clinical utility of cell-free and circulating tumor DNA in kidney and bladder cancer: a critical review of current literature. Eur Urol Oncol 2021;4:893-903.
23. Mouliere F, Robert B, Arnau Peyrotte E, et al. High fragmentation characterizes tumour-derived circulating DNA. PLoS One 2011;6:e23418.
24. Hindson BJ, Ness KD, Masquelier DA, et al. High-throughput droplet digital PCR system for absolute quantitation of DNA copy number. Anal Chem 2011;83:8604-10.
25. Taly V, Pekin D, Benhaim L, et al. Multiplex picodroplet digital PCR to detect KRAS mutations in circulating DNA from the plasma of colorectal cancer patients. Clin Chem 2013;59:1722-31.
26. Hinohara K, Polyak K. Intratumoral heterogeneity: more than just mutations. Trends Cell Biol 2019;29:569-79.
27. Pribluda A, de la Cruz CC, Jackson EL. Intratumoral heterogeneity: from diversity comes resistance. Clin Cancer Res 2015;21:2916-23.
28. De Mattos-Arruda L, Weigelt B, Cortes J, et al. Capturing intra-tumor genetic heterogeneity by de novo mutation profiling of circulating cell-free tumor DNA: a proof-of-principle. Ann Oncol 2018;29:2268.
29. Pantel K, Alix-Panabières C. Liquid biopsy and minimal residual disease - latest advances and implications for cure. Nat Rev Clin Oncol 2019;16:409-24.
Cite This Article
How to Cite
Download Citation
Export Citation File:
Type of Import
Tips on Downloading Citation
Citation Manager File Format
Type of Import
Direct Import: When the Direct Import option is selected (the default state), a dialogue box will give you the option to Save or Open the downloaded citation data. Choosing Open will either launch your citation manager or give you a choice of applications with which to use the metadata. The Save option saves the file locally for later use.
Indirect Import: When the Indirect Import option is selected, the metadata is displayed and may be copied and pasted as needed.
About This Article
Copyright
Data & Comments
Data
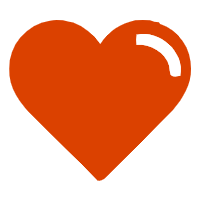
Comments
Comments must be written in English. Spam, offensive content, impersonation, and private information will not be permitted. If any comment is reported and identified as inappropriate content by OAE staff, the comment will be removed without notice. If you have any queries or need any help, please contact us at support@oaepublish.com.