Progress and challenges in heterocyclic polymers for the removal of heavy metals from wastewater: a review
Abstract
Water contamination by heavy metals has emerged as a global environmental problem. Their toxicity, non-degradability, and persistent nature make them a serious threat to human health, flora, and fauna. Therefore, several techniques have been developed for the removal of these pollutants from wastewater. Recently, linear aromatic polymers have received increasing attention for wastewater treatment due to the presence of various heterocyclic moieties containing electron-donating atoms such as nitrogen, oxygen, or sulfur on their backbone, which can be easily coordinated with metals, resulting in excellent affinity for heavy metals. This review article is specifically devoted to providing an overview of the various linear-architecture heterocyclic polymers that have been synthesized to be used as adsorbent phases for the removal of heavy metals from wastewater over the past fifteen years. The importance of incorporating heterocyclic units as efficient chelating sites for ion binding is highlighted. The adsorption mechanisms of different aromatic polymers are presented; their adsorption isotherms can primarily be modeled with the Langmuir model and their kinetics follow a pseudo-second-order kinetic model. The ways to improve the adsorption capacities of the linear aromatic polymers by increasing their specific surface area are discussed in the perspective paragraph, along with strategies to improve their reusability by choosing the proper acidic washing step.
Keywords
INTRODUCTION
In recent years, water pollution caused by heavy metal contamination has become a global concern[1]. Due to the rapid growth of industrialization and anthropogenic activities including metal plating, mining operations, tannery, batteries, chemical, automobile radiator manufacturing, and other industries, a dramatic increase in heavy metal pollution has occurred in the aquatic environment[2,3]. Extensive use of heavy metals in the above industries results in industrial wastewater rich in heavy metals that mix with lakes, rivers, oceans, and other drinking water sources, leading to water pollution[4,5]. There are various kinds of heavy metals, but the most predominant in industrial effluents are copper (Cu) (1.45 ± 1.22 mg/L), chromium (Cr) (65.81 ± 40.01 mg/L), lead (Pb) (21.28 ± 6.85 mg/L), iron (Fe) (90.52 ± 32.08), zinc (Zn) (62.90 ± 8.63 mg/L), cadmium (Cd) (25.65 ± 6.60 mg/L), mercury (Hg) (6.78 ± 3.36 mg/L), and nickel (Ni) (0.74 ± 0.80 mg/L)[6,7]. These heavy metal compounds are extremely toxic, persistent, non-biodegradable, and highly soluble in aqueous media[8,9]. They, therefore, rapidly penetrate into food chains and accumulate in living organisms, causing severe threats to humans and aquatic ecosystems[10,11]. Although the consumption of limited amounts of certain heavy metals is extremely essential for human beings due to their unique role in the human metabolic system, excessive intake can lead to harmful health effects[12]. For example, nickel (Ni) can be useful at non-hazardous levels; it functions as an enzyme activator and is involved in many important metabolic processes. However, the excessive ingestion of Ni may cause lung and kidney damage, gastrointestinal disorders, dermatitis, and breathlessness[13,14]. Zn is a versatile element that plays critical roles in various cellular processes, making it indispensable for all living organisms[15,16]. At excess levels, Zn can cause serious health problems such as neuronal and respiratory disorders, vomiting, nausea, anemia, and prostate cancer[17]. Although Cu is an essential metal for humans, it is also potentially toxic above supra-optimal levels[18]. Cobalt (Co) serves as a metal component of vitamin B12 (cyanocobalamin), but can be hazardous to the human body following excessive exposure[19]. Heavy metal levels in drinking water are strictly controlled by powerful organizations such as the World Health Organization (WHO) and the US Environmental Protection Agency (USEPA)[20] [Table 1].
Acceptable heavy metal levels according to WHO and USEPA
Heavy metals | WHO | USEPA |
Cd | 3 µg/L | 5 µg/L |
Hg | 6 µg/L | 2 µg/L |
Cr total | 50 µg/L | 100 µg/L |
Cu | 2,000 µg/L | 1,300 µg/L |
Pb | 10 µg/L | 15 µg/L |
Ni | 70 µg/L | 100 µg/L |
Fe | 1,000-3,000 µg/L | 300 µg/L |
Zn | 3,000 µg/L | 5,000 µg/L |
Therefore, efforts have been devoted to researching effective technologies for removing toxic metals from wastewater[5]. Numerous water decontamination techniques have been applied, including chemical precipitation[21], ion exchange[22], membrane filtration[23], and electrochemical processes[24,25]. However, these techniques are difficult to remove heavy metals in low concentrations[26]. Up to now, adsorption has been considered the best process for removing damaging metals from water resources, thanks to its higher efficiency, simplicity, ease of accessibility, cost-effectiveness, and environmentally-friendly nature[27,28]. The additional advantage of adsorption is that adsorbents can be easily regenerated using an appropriate desorption process[29-31]. Many adsorbents are employed to trap heavy metals in wastewater. The conventional adsorbents are activated carbons, activated clays, zeolites, and silica gels[32-37]. In recent decades, many efforts have been made to remove heavy metals, using several types of organic linear polymers bearing heterocyclic moieties in their main or lateral chains. Heterocyclic polymer based-adsorbents are one of the best alternatives for heavy metal adsorption due to the presence of heteroatom groups on their chains that can be chelating sites for metals, thus making the resulting polymers effective adsorbent phases to remove different metals from wastewater. Moreover, the incorporation of heteroaromatic rings on the polymer chains is the most common approach for increasing the chemical and thermal stability of the resulting polymers and their hydrophilicity, which is a necessary property to extract inorganic pollutants from water media[38,39]. The recent developments in synthesized heterocyclic polymers-based adsorbents for the extraction of various toxic metals from wastewater are reviewed here. This review was based on the querying of the scientific database (Web of Science, Scopus, and Google Scholar) through the main keywords: Adsorption, heterocyclic linear polymers, polyimides (PIs), polyamides, heavy metals, wastewater, and through a selection of the published papers in the last 15 years. To our knowledge, no other review specifically oriented to the use of heterocyclic polymers for the removal of heavy metals from wastewater has been published until now. The published reviews, such as the one by Malik et al., have cited all types of adsorbents used for heavy metal removal, but only a few examples of polymers have been mentioned[5].
HEAVY METALS AND THEIR IMPACT ON HUMAN HEALTH
Heavy metals are part of the natural environment, and their traces are essential for human health. However, their excessive use on an industrial scale generates considerable pollution in water systems, due to their discharge through industrial wastewater, posing a severe threat to the ecological environment and public health. Many heavy metals, including Hg, Cd, Pb, Zn, Cr, As, Cu, Fe, Mn, Ag, Co, and Ni, have been identified as the most abundant in water and soil[40]. These metals are highly soluble in water and easily accumulate in plants and the human body through the food cycle[41]. Long-term exposure to heavy metals leads to serious diseases and an increased risk of some cancers[42,43]. Exposure to high levels of Hg affects the central nervous system and can damage the functionality of the brain, kidneys, and liver[44-46]. Cd is known as a carcinogenic metal and can cause kidney dysfunction, cardiovascular diseases, lung cancer, hypertension, and testicular atrophy[47,48]. Pb is another heavy metal with negative effects on human health, such as Alzheimer’s disease, kidney damage, mental retardation, reproductive system dysfunction, and cancers[49,50]. Chronic exposure to Zn has been reported to cause fertility disorders, cancer, and cholesterol imbalance[51,52]. Arsenic exposure has been linked with heart disease, bronchitis, dermatitis, mental disorders, and lung and skin cancers[53,54]. Cr exists as a trivalent ion [Cr(III)] and as a hexavalent ion [Cr(IV)], which is the form most harmful to humans, plants, and animals. It can cause lung tumors, digestive tract cancer, mutagenicity and embryotoxicity, hemorrhage, and skin irritation[55,56]. Cu toxicity has been associated with kidney disorders, vomiting, diarrhea, gastroenteritis, liver damage, and even death[57,58]. Excessive silver consumption causes serious toxicological issues, such as changes in blood cells, and liver and kidney damage[59].
ADSORBENTS
Several adsorbents have been used for the adsorption method that can be sorted into three main families: industrial adsorbents, bioadsorbents, and polymeric adsorbents. Industrial adsorbents generally present specific surface areas greater than 100 m2/g, up to 1,000 m2/g. The most popular industrial adsorbents are activated carbons, zeolites, silica gels, and activated clays. Approximately 150.000 t/year of activated zeolites, 400.000 t/year of activated carbons, 400.000 t/year of activated clays, and 25.000 t/year of silica gels have been produced[60]. The most popular industrial adsorbents are activated carbons, zeolites, silica gels, and activated clays, all of which demonstrate excellent adsorption capacities for metal removal. Zeolites consist of micropores and aluminosilicate compounds that form a network of SiO4 and AlO4- tetrahedra, linked by common oxygen atoms[61]. These materials present a large specific surface area, high cation exchange capacity, non-emission of toxic compounds, and excellent heat resistance, which make them suitable for the removal of undesired heavy metals[62]. Due to the low ion exchange capacities of natural zeolites, chemical or physical methods are frequently used to enhance their adsorption performance[63]. Álvarez et al. compared the adsorption efficiency of activated zeolite with that of natural zeolite for removing Cr (VI)[64]. They reported that adsorption capacity depended on the type of activation and decreased in the following order: activated zeolite with NaOH (82%) > natural zeolite (34%) > activated zeolite with HCl (13%). Mehdi et al. also prepared an activated zeolite as an adsorbent material for Ni2+ removal from an aqueous solution[65]. The study revealed that the adsorption process was temperature-dependent. The maximum adsorption capacity expected from Langmuir isotherm was found to be 28.79 mg/g at 20 °C. In addition, the values of Freundlich constant n relating to the adsorption intensity were increased from 2.52 to 3.11, with increasing temperature of solution from 20 to 60 °C, indicating better adsorption at a higher temperature.
Adsorption onto activated clay can also constitute an efficient technique for decontaminating water polluted by toxic metals. Khalfa et al. studied the removal of several metals Cu2+, Pb2+, Zn2+, and Cd2+ using natural and activated clay to assess the effectiveness of these abundant materials in treating wastewater[66]. The authors reported that the heavy metal adsorption properties of natural and activated clay were Pb2+ > Cu2+ > Cd2+ > Zn2+. They also noted that activated clay has eliminated greater amounts of metals than pure clay. In fact, after sulfuric acid treatment of clay, the adsorption capacity of Pb2+, Cu2+, Cd2+, and Zn2+ was found to increase from 29.11, 22.30, 21.93, and 20.86 mg/g to 50.94, 33.66, 26.74, and 26.44 mg/g, respectively.
Activated carbon is the most commonly used and widely produced adsorbent for wastewater treatment. It has recently received significant interest for the removal of heavy metals due to the large surface area, suitable surface functional groups, and appropriate pore diameter[68]. Numerous researchers have examined the adsorption capacity of activated carbon as a function of changes in preparation factors [Table 2]. It has been shown that the nature of raw material, the preparation conditions (temperature, heating rate, and duration), and the type of activation method (physical or chemical) have a significant influence on the physicochemical properties of activated carbon and therefore on adsorption performance[69-74].
Adsorption behavior of activated carbon prepared under different conditions
Used raw material | Temperature of preparation (°C) | Time of preparation (min) | Surface area (m2/g) | Heavy metal | Adsorption capacity (mg/g) | 1/n(a) | Ref. |
Rape straw | 300 | 120 | 699.9 | Pb (II) | 253.2 | 0.181 | [69] |
Sugar beet bagasse | 450 | 120 | 748 | Cr (VI) | 52.8 | 0.592 | [70] |
Rice straw | 400 | 240 | 4.39 | Cd (II) | 37.14 | - | [71] |
Gingko leaf | 800 | 90 | 310 | Cu (II) | 59.90 | 0.3785 | [72] |
Rice husk | 600 | 10 | 768.98 | Hg0 | 0.016 | - | [73] |
Pine bark | 600 | 24 | - | Cd (II) | 85.8 | 0.3364 | [74] |
However, the use of activated carbon is limited by its high production cost, poor selectivity, difficult functionalization, and low regeneration. Its regeneration requires high energy (500-900 °C) with a 10%-15% loss of the carbonaceous material and a limited adsorption capacity. The recovery of the adsorbed heavy metals decreases the process cost; nevertheless, eluents should not damage the adsorbent and should be cost-effective and eco-friendly. Diluted HCl solutions were used to recover the heavy metals[75]. Therefore, the adsorption onto economic sorbents such as bio-based materials has been the subject of numerous studies, due to their availability, inexpensiveness, biodegradability, biocompatibility, and renewability. These parameters have a great impact on environmental practices. There are several agricultural by-products, such as banana peel[76], green tea waste[77], rice husk[78], and treated olive pulp[79], that have been employed as bio-adsorbents to remove heavy metals in water.
Polysaccharides are the most abundant carbohydrate and possess excellent properties for use as bio-based adsorbents for metals[80-82]. These natural polymers, such as cellulose, carrageenan, alginate, starch, cyclodextrin, chitin, and its derivative chitosan, have obtained vast attention as practical and low-cost adsorbents as opposed to activated carbons. Many attractive properties of polysaccharides, such as their specific structure, high reactivity, chemical stability, and excellent selectivity for metals, have made them such smart alternative adsorbents. Moreover, the chemistry of their surface can be modified by grafting functional groups onto chains to acquire new adsorption properties. Yu et al. proposed the grafting of amino-terminated hyperbranched polyamide (HP) onto the surface of cellulose-bearing aldehyde groups for efficient removal of metals[83]. This material shows excellent adsorption performance for Cu2+ with an adsorption capacity of 138 mg/g. Guleria et al. developed a poly-(acrylamide-co-acrylic acid) functionalized cellulose adsorbent to improve its heavy metal adsorption performance[84]. Triazole groups have also been grafted onto cellulose chains by Yin[85] to eliminate Gd(III) and by Mahalakshmi[86] to adsorb efficiently Pb2+, Co2+, Ni2+, and Cd2+. This study revealed that incorporating heteroatoms as chelating units in the cellulose backbone plays a major role in the adsorption process with the coordination reaction between nitrogen atoms in triazole and metals.
Polymeric adsorbents including ion exchange resins, molecularly imprinted polymers (MIPs), and porous organic polymers (POPs) have been utilized extensively in the remediation of metals from water[87-89], but the usage of linear organic polymers as adsorbents is less reported. Over the last few years, heterocyclic polymer-based adsorbents, such as polypyrroles, polyamides, PIs [Table 3], have received increasing attention from researchers because of their potential applications in the uptake of various heavy metals and the fact that the incorporation of different functional groups in polymer matrices enables them to be used as adsorbents capable of efficiently absorbing numerous organic and inorganic pollutants. In addition, these polymers can easily be regenerated under mild conditions.
Heavy metal ions adsorption using various linear aromatic polymers
Polymer adsorbent | Repetitive unit | Metal ion | Adsorption capacity (mg/g) or % | Affinity Parameter (1/n) | Ref. |
Quinoxaline-based PTIs | ![]() | Cr2O72-, Pb2+, Hg2+, Cd2+, Zn2+, Co2+, Mn2+, Cr3+ | 28%, 33%, 40%, 44%, 50%, 60%, 85%, 89% (pH = 8) | - | [90] |
Phenoxy quinoline-based PTAIs | ![]() | F-, Cr2O72-, Cr3+, Cd2+, Pb2+, Hg2+ | 9.50, 8.56, 6.60, 6.66, 7.97, 8.76 mmol/g (pH = 3). 5.96, 4.99, 7.99, 7.90, 8.94, 9.2 mmol/g (pH = 10) | - | [91] |
Phenoxy quinoline-based PTIs | ![]() | F-, Cr2O72-, Cr3+, Cd2+, Pb2+, Hg2+ | 8.91, 7.55, 5.0, 4.2, 5.5, 5.71 mmol/g (pH = 3) 5.0, 4.0, 6.1, 7.0, 8.38, 8.8 mmol/g (pH = 10) | - | [92] |
Polyimides containing imidazole and xanthene pendants | ![]() | Hg2+, Cr3+, Cd2+, Co2+, Pb2+ | 37.9, 32.65, 28.6, 21.15, 19.80 mmol/g | - | [93] |
Polyimides containing pyridine and xanthene pendants | ![]() | Cr (VI) | 91% | - | [94-96] |
Porphyrin-based PIs | ![]() | Cd2+, Hg2+ | 28.59, 27.73 mmol/g | - | [97,98] |
Biosourced polyimides | ![]() | Pb2+, Ni2+, Cd2+, Hg2+ | 0.726, 0.818, 0.488, 0.084 | 0.096, 0.064, 0.102, 0.181 | [99] |
PXIs | ![]() | Cd2+, Pb2+, Cu2+,Ni2+, Co2+, Cr (VI) | 57.3%, 47%, 45.2%, 42%, 41.1%, 39% | - | [100] |
Polyimides bearing1,3,4-oxadiazole and pyridine units | ![]() | Co2+, Ni2+ | 110.4, 100.5 mmol/g | - | [101] |
Sulfonated copolyimides | ![]() | Pb2+ | 99% | - | [102] |
Chlorobenzylidine-based polyamides | ![]() | Pb2+, Cr3+ | 274.6, 255.2 mmol/g | - | [103] |
PAMAs | ![]() | Cu2+, Cd2+, Pb2+ | 470.7, 462.3, 452.1 mmol/g | 0.345, 0.393, 0.413 | [104] |
Pyridine-containing polyamides | ![]() | Cu2+, Pb2+ | 389.9, 403.1 mmol/g | - | [105] |
1,2,4-triazole-based polyamides | ![]() | Hg2+ | 99% | - | [106] |
Imidazole-based polyimides Imidazole-based polyamides | ![]() ![]() | Cr3+, Co2+, Cd2+, Hg2+, Pb2+ | 38.45, 26.20, 20.5, 18.40, 13.30 mmol/g 43.90, 30.80, 25.10, 21.60, 19.60 mmol/g | - | [107] |
Sulfonated polyamides | ![]() | Pb2+, Hg2+ | 11.87, 5.17 mmol/g | - | [108] |
PAS | ![]() ![]() | Hg2+ Pb2+ | 47.95 mmol/g 714 mmol/g | 0.14 0.059 | [109] [110-113] |
Poly(amide-hydrazide-imide)s | ![]() ![]() | Pb2+, Cd2+, Cu2+, Zn2+, Hg2+ Pb2+, Cd2+, Cu2+, Zn2+, Hg2+ | 0.99, 075, 0.47, 0.31, 0.037 mmol/g 1.70, 0.71, 0.54, 0.29, 0.43 mmol/g | - | [114] [115] |
Poly(2-aminothiazole) | ![]() | Ag+ | 336.98 | 0.233 | [116] |
1,2,3-triazole based-poly(ionic liquid) | ![]() | Cr (VI) | 13.545 | 0.289 | [117] |
1,2,3- triazole containing polystyrene | ![]() | Mg2+, Zn2+ | 3, 30% | - | [118] |
Polyazomethines | ![]() ![]() | Co2+, Cu2+, Au3+ Ni2+, Cu2+, Co2+ | 21.28, 31.25, 35.71 25.64, 15.38, 31.25 | - | [119] [120] |
Poly(etherphosphine)s | ![]() | Pb2+, Cd2+, Hg2+, Ni2+ | - | - | [121] |
Diethylphosphonate-containing poly(arylene ether nitrile)s | ![]() | Pb2+, Cd2+, Hg2+, Ni2+ | - | - | [122] |
HETEROCYCLIC LINEAR POLYMER-BASED ADSORBENTS
A review of recent adsorbents used in metal removal from contaminated wastewater is presented here, with a focus on linear functional polymeric adsorbents. These polymers can be produced from different polymerizations of heterocyclic monomers. Furthermore, the impact of different factors such as the pH of the solution, the adsorbent dosage, the initial concentration of heavy metals, and the contact time on the adsorption capacity of polymers-based adsorbents are studied.
PIs
PIs are one of the most important aromatic polymer classes, offering excellent thermal stabilities, sufficient chemical resistance, and high mechanical and electrical properties. However, they present very poor hydrophilicity, and therefore, many efforts have been undertaken to incorporate heterocyclic functional groups into their backbone or side groups to generate new PIs with enhanced thermal properties and improved chelating properties.
Among various heterocycle structures, 1,2,4-triazole has been the subject of numerous studies, mainly due to its stability under diverse conditions and the well-established role of triazole units as binding sites for metals. Therefore, insertion of the triazole ring into the main chains produces materials with high hydrophilicity, enabling them to interact with metals. Bazzar et al. synthesized a series of PIs bearing 1,2,4-triazole moiety in the principal chain and quinoxaline as a pendant group, according to a two-step procedure that involves the condensation of diamines with dianhydrides followed by the imidization process[90]. The obtained poly(triazole-imide)s were used as adsorbents of Cr3+, Co2+, Zn2+, Pb2+, Cd2+, Hg2+, CrVI, and Mn2+ individually or in mixtures, at a range of pH values (1-14). The removal percentage of metals was increased in the order of Cr3+ > Mn2+ > Co2+ > Zn2+ > Cd2+ > Hg2+ > Pb2+, which is contrary to the order of their ion radii. The maximum adsorption of 98% was given to the smallest metal ion (Cr3+), while 35% removal was observed for the largest metal ion (Pb2+) [Figure 1A].
Figure 1. (A) The quantity of adsorbed metals (Qt) and adsorption efficiency (%R) at pH = 8 by poly(triazole-imide); (B) effect of pH variation on the adsorption amount of Cr3+ cation; (C) effect of pH variation on the amount of adsorption of Cr2O72- anion. From[90] with permission.
A series of solutions at different pH values ranging from 1.0 to 14.0 was prepared to study the effect of initial solution pH on metal ion removal. As shown in Figure 1B, the percentage of Cr3+ removal was low in the pH zone between 1 and 5, and increased with increasing pH, reaching a maximum of 98% at a basic pH of 10. While the adsorption efficiency of Cr2O72- anion was 80% at pH 1.0 and decreased as pH increased [Figure 1C]. This finding can be explained by the fact that in the acidic pH range, nitrogen atoms of the triazole and quinoxaline cycles are protonated, resulting in a repulsive interaction between the positive ions and the positively charged polymer surface that reduces the adsorption of the Cr3+ cation. On the other hand, the negative-charged Cr2O72- is electrostatically attracted to positive bonding groups on the PI surface. The solution pH is an important parameter as it can affect the adsorbent’s functional group ionization and can determine the attractive or repulsive interaction between the adsorbent and the metals to enhance or reduce adsorption efficiency. Ghaemy et al. also reported the influence of pH on metal adsorption ability using triazole-based poly(amide-imide) bearing a pendant phenoxy quinoline unit[91]. The polycondensation reaction was performed between a diimide-diacid and three commercial diamines in the presence of thiamine pyrophosphate as an activator in NMP (N-méthyl-2-pyrrolidone)/Pyridine mixture containing LiCl and CaCl2. At acidic media pH = 3, the highest extraction percentages of 95% and 85% were found for F- and Cr2O72- anions, respectively, and the adsorption of Cr3+ and Cd2+ cations showed the lowest value of 66% at this pH. The adsorption study revealed that polymer removal performance depended mainly on the structure and hydrophilic character of the diamine moiety in the polymer backbone. The highest adsorption percentage was observed with the polymer containing two hydrophilic triazole rings in the repeating unit, increasing its hydrophilic character and adsorption capacity. The same authors[92] reported the design of another family of phenoxy quinoline-based poly(triazole-imide)s for the efficient removal of metals. The triazole and phenoxy quinoline groups were identified as effective chelating and host units for heavy metals. According to this research, structural modification of PI by incorporating heterocyclic rings can be an interesting approach to improving their adsorption capacity.
Flexible ether linkages, imidazole rings, and xanthene as a pendant group have been successfully added to PIs by Amininasab et al.[93]. The use of Imidazole heterocyclic rings and their derivatives is advantageous due to their high electron affinity and good thermal stability. The prepared PIs showed an enhanced adsorption capacity for adsorbing heavy metals due to the increased presence of –NH and –O groups along the adsorbent’s backbone. The adsorption capacities for Hg2+, Cr3+, Cd2+, Co2+, and Pb2+ were 37.90, 32.65, 28.60, 21.15, and 19.80 mg/g, respectively. For efficiently removing Cr (VI) from wastewater, PIs were functionalized with pyridine and xanthene groups[94].
The pyridine heterocyclic ring has been reported to improve the processability, solubility, and adsorption performance of PIs due to its aromaticity and polarizability resulting from nitrogen heteroatoms[95], which are recognized as the main adsorption sites and effective binding sites for heavy metals to form metal complexes which are known as the main adsorption site and as an effective binding site of heavy metals to form a metal complex[96]. The adsorption behavior of these polymers at different Cr (VI) concentrations (1, 5, and 10 mg/L) showed that the adsorption capacity (91%) was higher when the concentration of hexavalent Cr in aqueous solution was 5%. Rafiee et al., in turn, described the development of new PIs having porphyrin units utilizing four tetracarboxylic dianhydrides and tetramine through a two-step imidization technique for the adsorption of Cd2+ and Hg2+[97]. Porphyrins are regarded as an attractive class of heterocyclic materials with a high capacity to bind with a diverse array of metals and create stable complexes. The nitrogen atoms in the tetrapyrrolic core, due to their electron-donating properties, can serve as ligand sites to adsorb various metals, allowing their efficient extraction[98]. Adsorption tests were performed by dissolving 5 mg of a single PI in 25 mL of individual aqueous metal ion solutions at pH 7 and stirring for 3 days. The results showed that the utilized PI had an excellent adsorption capacity toward Cd2+ > Hg2+.
Bio-sourced coPIs were used as adsorbent films which is similar to the order
f Pb2+, Ni2+, Cd2+, and Hg2+ from aqueous solution[99]. These polymers were synthesized by combining, from 100% to 0%, three monomers (isosorbide-based dianhydride, petroleum-based dianhydride, and cardo diamine). It was noted that adsorption efficiency increased as the proportion of isosorbide moieties in the PI backbones increased. Thus, the homopolyimide, obtained from 100% of biobased dianhydride and diamine, exhibited the highest adsorption efficiency toward metals. This rise is mainly due to the incorporation of isosorbide into the polymer structure, which can generate attractive hydrophilicity and wettability, increasing their tendency to remove contaminants from water. Comparing the adsorption capacity of metals on this polymer, the order was as follows: Pb2+ ≈ Ni2+ > Cd2+ > Hg2+. Based on the 1/n values, the adsorption of Ni2+ (0.064) and Pb2+ (0.096) is deemed to be more advantageous, while the adsorption of Hg2+ (0.181) is less favorable. In addition, the proposed adsorption mechanism was mainly based on oxygen-heavy metal interactions [Figure 2].
Figure 2. The metal-polyimide complex formed via oxygen-heavy metal interaction. From[99] with permission.
Lakouvaj et al. also investigated the extraction of metals including Cr (VI), Co (II), Ni (II), Cu (II), Pb (II), and Cd (II) from aqueous solutions using a new class of poly(xanthone-imide)s (PXIs)[100]. The authors revealed that the adsorption efficiency (%) increased in the order of Cd2+ > Pb2+ > Cu2+ > Ni2+ > Co2+ > Cr(VI), which is similar to the order of their ionic radii. They stated that the carbonyl functional groups in PI chains act as coordination sites for chelation with metals [Figure 3].
Figure 3. Chelation of metals with PXIs[100]. PXIs: Poly(xanthone-imide)s.
Mansoori et al., in a similar research, reported the synthesis of PIs containing 1,3,4-oxadiazole and pyridine moieties for removing Co2+ and Ni2+[101]. It has been demonstrated that variations in solution pH impact the coordination capabilities of PIs toward metals. Consequently, the highest metal uptake capacities for Co (II) and Ni (II) were 110.4 and 100.5 mg/g, respectively, at pH 7.0. This finding was reported by Manzoor et al. It was shown that variations in solution pH affect the coordination capacities of PIs toward metals[102]. Thus, the highest metal uptake capabilities of Co2+ and Ni2+ were 110.4 and 100.5 mg/g, respectively, at pH 7.0. Manzoor et al. prepared two aromatic sulfonated copolyimides (SPIs), FBM and OBM, using two distinct dianhydrides FDA [4,4’-(hexafluoroisopropylidene)-diphthalic anhydride] and ODPA (4,4’-oxydiphthalic anhydride), along with stoichiometric amounts of sulfonated [BDSA (Benzidine-2,2’-disulfonic acid)·TEA (triethylamine)] and non-sulfonated [MDA (4,4′-methylenedianiline)] diamines as comonomers, aiming for effective Pb adsorption from aqueous solutions[102]. They reported that incorporating anionic sulfonate groups into the PI backbone improved hydrophilicity, resulting in enhanced Pb2+ adsorption.
The authors employed quantum chemical simulations using Molecular Operating Environment software (MOE) to identify the structural factors affecting the sorption efficiency of Pb2+ onto SPI adsorbents. It was observed that OBM has a hydrophilic surface area larger than that of FBM, as shown in Figure 4. The enhanced hydrophilicity of the OBM copolyimide contributes to its increased adsorption capacity, which is consistent with experimental results, with data showing higher adsorption of Pb ions on the OBM surface (99%) compared to FBM (30%).
Figure 4. Hydrophobic regions (depicted as green dots) and hydrophilic regions (represented by blue and purple areas) of two sulfonated aromatic copolyimides, the sulfonated FBM (A) and non-sulfonated OBM (B) complex with Pb. From[102] with permission.
Polyamides
Heterocyclic polyamides represent one of the most important classes of high-performance materials, which are ideal candidates for use as heavy metal ion adsorbent from aqueous solutions. Significant attention has been drawn to the synthesis of polyamides containing –CONH–, –CSNH–, –N=CH–, and heterocyclic groups that can serve as metal-complexing ligands. The presence of these chelating functionalities in an aromatic polyamide backbone is expected to enhance metal ion adsorption capacity and stability for reuse.
Ravikumar et al. synthesized aromatic polyamides and polythioamides incorporating pendant chlorobenzylidine rings via direct polycondensation of a dicarboxylic acid with diamines and thioamines, respectively, for the removal of toxic metals from water[103].
They reported that the amide, carbonyl, and azomethine groups in the polymer backbone enhanced the adsorption capacity of Pb2+ and Cr3+ from water. The highest adsorption capacity for Pb2+, 274.6 mg/g, was achieved at pH 10, while the maximum adsorption for Cr3+, 255.2 mg/g, occurred at pH 6.
Murugesan et al., on the other hand, examined the elimination of Pb+2, Cu+2, and Cd+2, using aromatic poly(azomethine amide)s[104]. Their results showed that the removal of metals varied with pH, peaking at
Albukhari et al. synthesized a series of four heteroaromatic sulfur-containing polyamides by polycondensation of diamine with diacid chlorides for the selective removal of heavy metals from wastewater[109]. The obtained polymers demonstrated a remarkably high selectivity for Hg (II) ions, with the maximum adsorption capacity reaching 47.95 mg/g by the Langmuir model. The effect of pH (ranging from 1 to 9) on the adsorption of Hg+2 was also studied. It has been reported that the highest percentage of Hg+2 extraction, 98.75%, was achieved at pH 1. This increase was explained by the electrostatic attraction forces that can occur between protonated sites formed at pH 1, such as secondary amine groups and carbonyl groups in the polymer backbones, with the negatively charged species including HgCl4-2, neutral HgCl2, and another chloroanionic complex HgCl3-1. In another study, Rezania et al. prepared a polyamide-sulfide (PAS) incorporating pyridine and thiazole heterocycles for Pb removal from water[110]. They observed that the synthesized polymer is an excellent adsorbent of Pb(II) ions, demonstrating a significant adsorption capacity of around 714 mg/g.
Replacing polyamides with copolyamides such as poly(amide-imide)s (PAIs) may be beneficial for increasing the polarity of the polymer chain to extract heavy metals with more affinity. Due to the presence of both amide and imide units in their polymer repeating units, PAIs exhibit properties that are different from those of polyamides and PIs. As a result, this class of polymers provides a good combination of excellent thermal stability, processability, and high uptake of heavy metals[111-113].
The adsorption of PAIs was enhanced by incorporating hydrazide functional groups in their backbone. This improvement is attributed to the polar characteristics of the hydrazide group (–CO–NH–NH–CO–), which readily engages with metals to create complexes[114]. These heterocyclic polymers were prepared by polycondensation of a diacid monomer containing imidic rings with various dihydrazides for the extraction of heavy metal cations such as Pb, Cd, Cu, and Zn. Among heavy metals, Pb demonstrated the highest adsorption capacity at 0.99 mmol/g, while Cd ions were adsorbed with a capacity of 0.75 mmol/g. A comparative study was carried out using another family of poly(amide-hydrazide-imide) obtained from the reaction of an acid chloride-anhydride monomer with dihydrazides, which resulted in more efficient adsorption of Pb2+ (1.70 mmol/g)[115].
Other heterocyclic polymers
Powerful adsorption of silver ions was obtained utilizing a poly(2-aminothiazole)P-2AT prepared via the polymerization of 2-aminothiazole with benzoyl peroxide (BPO) as an initiator in 1,4-dioxane at 80 °C for 24 h[116]. The adsorption tests were performed at different pH levels (3-6), contact times (15-90 min), and initial Ag+ concentrations (100-1,200 mg/L) to study the effect of these parameters on adsorption capacity. It revealed that adsorption efficiency increased with the increasing initial concentrations and the contact time. The maximum adsorption capacity of Ag+ on P-2AT was found to be 336.98 mg/g for 1,200 mg/L Ag (I) solution at pH 5 after 90 min of contact. An attractive heavy metal adsorbent based on a poly(ionic liquid) bearing 1,2,3-triazole moiety was developed by Puguan and Kim for Cr (VI) extraction[117]. It was prepared by Cu-catalyzed cycloaddition CuAAC of α-azide-ω-alkyne monomer in the presence of CuIP (OEt)3 followed by quaternization and anion exchange. The Langmuir isotherm model described the adsorption of Cr (VI) on the poly(ionic liquid) as monolayer adsorption with an adsorption capacity of 13.545 mg/g at room temperature and pH 2. The adsorption was the result of van der Waals and electrostatic forces between the amines in the triazole ring and the dissociated Cr (VI) in water. The 1,2,3-triazole unit has also been grafted into polystyrene chains [Figure 5] to be tested as sorbents for the removal of Mg and Zn present in wastewater[118]. These polymers showed a low selectivity for Mg, with an extraction percentage of below 3%, while for Zn, the removal percentage was around 30%.
Figure 5. Synthetic Scheme of 1,2,3-Triazoles-Based Polystyrene and its application in the Extraction of Zn and Mg from Wastewater. From[118] with permission.
Qureshi et al. synthesized polyazomethine through a polycondensation reaction of dialdehyde and diamine for the adsorption of Co2+, Cu2+, and Au3+ from wastewater[119]. The sorption process followed the Langmuir monolayer adsorption model, and the adsorption capacities for Co2+, Cu2+, and Au3+ were 21.28, 31.25, and 35.71 mg/g, respectively. The results indicated a chemisorption/ion-exchange nature of adsorption. The authors varied several parameters, including initial ion concentration (10-50 mg/L), pH levels (2-8), the quantity of polymer adsorbent (10-50 mg), and the contact time (10-180 min), to obtain maximum adsorption capacity from the optimum adsorbent. The ideal conditions obtained for the highest adsorption capacity of Co2+, Cu2+, and Au3+ are at a pH value of 8 for Co2+ and Cu2+ and 2 for Au3+. The corresponding adsorption capacities were 4.32, 51.8, and 41.32 mg/g, respectively.
Likewise, a polyazomethine derived from another diamine[120] was also prepared and used for the efficient extraction of Ni2+, Cu2+, and Co2+. This material removed up to 77% of Ni2+, 98% of Cu2+, and 72% of Co2+ from wastewater. Chabbah et al. developed phosphorous polymers for the detection of Pb2+, Cd2+, Hg2+, and Ni2+ heavy metals[121,122]. Their results showed that the synthesized polymers display a performing ability for the decontamination of water.
ADSORPTION MECHANISMS
The Langmuir adsorption model assumes that a polymer has a fixed number of binding sites. All the binding sites show the same affinity for the adsorbent. Therefore, each site adsorbs only one molecule and forms a single monolayer.
The linear form of the Langmuir model can be written as:
1/qe = 1/bqm (1/Ce) + 1/qm (Eq. 1)
Where qe (mg/g) is the amount of heavy metals adsorbed onto the polymer at equilibrium, Ce (mg/L) is the equilibrium concentration, qm (mg/g) denotes the maximum adsorption capacity, and b (L/mg) is the Langmuir constant related to free energy. The Langmuir constants qm and b were calculated from the slope and the intercept of the Langmuir plot of 1/qevs. 1/Ce.
The linear form of the Freundlich model is given as:
lnqe = 1/n lnCe + lnkF (Eq. 2)
Where qe (mg/g) represents the amount of heavy metals adsorbed per unit mass of adsorbent, Ce (mg/L) is the equilibrium concentration of heavy metals, and n and kF are Freundlich constants. These constants can be determined from the linear plot of lnqevs. lnCe.
The Redlich-Peterson isotherm combines Langmuir and Freundlich models. At higher concentrations, it approximates the Freundlich model, while at lower concentrations, it aligns with the Langmuir equation. The equation is given as:
qe = (KRCe)/(1 + aRCeb) (Eq. 3)
where KR is the Redlich-Peterson isotherm constant (L/g), αR is the Redlich-Peterson isotherm constant
The Temkin isotherm model contains a factor that explicitly takes into account the adsorbing species - adsorbate interactions. This model assumes the following conditions: (1) the heat of adsorption of all the molecules in the layer decreases linearly with the coverage due to adsorbent-adsorbate interactions; and (2) the adsorption is characterized by a uniform distribution of binding energies, up to some maximum binding energy. The derivation of the Temkin isotherm assumes that the fall in the heat of sorption is linear rather than logarithmic, as implied by the Freundlich equation. The Temkin isotherm has commonly been applied in the following form:
qe = Bln (ACe) (Eq. 4)
where B = RT/b and A is Temkin constant.
The kinetics of heavy metal adsorption onto the different fabricated polymers were analyzed at various time intervals using the pseudo-first-order and pseudo-second-order kinetic models. The pseudo-first-order kinetic model is expressed as:
ln (qe - qt) = -k1t + lnqe (Eq. 5)
where qt and qe (mg/g) are the amount of heavy metals adsorbed at time t (min) and equilibrium time, respectively. The parameter k1 (min-1) denotes the rate constant of the pseudo-first-order kinetic model. The rate constant of the pseudo-first-order kinetic model and qe values are derived from the slope and the intercept of the linear plots of ln(qe - qt) vs. time (min), respectively. The pseudo-first-order kinetic model assumes that one heavy metal ion is sorbed onto one sorption site on the surface of a polymer.
The pseudo-second-order kinetic model can be written as:
1/qt = t/qe + 1/k2qe2 (Eq. 6)
where k2 (g/mg/min) is the rate constant of the pseudo-second-order kinetic model. This model assumes that one heavy metal ion is sorbed onto two sorption sites on the surface of an adsorbent. The rate constant of the pseudo-second-order kinetic model and qe are estimated from the intercept and the slope of the linear plots of t/qtvs. time (min), respectively.
The adsorption isotherms were best fitted with Langmuir model for the adsorption of heavy metals on biosourced PI[99], polyamide sulfide[109], 1,2,3-triazole based-poly(ionic liquid)[117], and polyazomethines[119]. Redlich-Peterson model allowed the best fitting of the adsorption isotherms of heavy metals on poly(azomethine amide)s[104]. This point suggests that the polymer surface presents a homogeneous surface with specific adsorption sites.
The pseudo-second-order kinetic model allowed the best fitting of the adsorption of heavy metals on poly(azomethine amide)s[104], 1,2,3-triazole based-poly(ionic liquid)[117], and polyazomethines[119], suggesting that the rate-limiting step of heavy metal adsorption is mainly chemisorption process.
PERSPECTIVES AND CHALLENGES
The main factors characterizing the adsorbent qualities are its affinity, represented by the factor 1/n (with a lower value indicating higher affinity), and its adsorption capacity. Some quantitative data for conventional adsorbents can be found in the literature [Table 4].
Comparison of the characteristics of heterocyclic polymers to other popular heavy metal absorbents
Adsorbent | Heavy metal | Adsorption capacity mg/g | Affinity (1/n) | Ref. |
Zeolite | Ni | 28.79 | 0.39 | [65] |
Activated clay | Cr | 18.15 | 0.56 | [71] |
Fe | 39.80 | 2.80 | [71] | |
Activated carbon | Pb | 253.2 | 0.181 | [69] |
Cd | 85.8 | 0.3364 | [74] | |
Biosourced polyimides | Ni | 0.064 | [99] | |
Polyamide-sulfides | Pb | 0.059 | [110] | |
Poly(etherphosphine) | Pb | 1 | [121] | |
Poly(azomethine amide) | Pb | 403.1 | [105] | |
Pb | 452.1 | [104] | ||
Functionalized amino-rich PI-PEI fibers | Cr | 50 | [124] | |
Electrospun Fe3O4/MWCNTs/polyamide 6 nanofibers | Pb | 49.3 | [125] | |
Electrospun PA6/CaO nanofibers | Pb | 39 | [126] | |
Cr | 33 |
For zeolites[65], 1/n is equal to 0.39 and the adsorption capacity is 28.79 mg/g for Ni (II) ions at 20 °C. For activated clays[71], 1/n is equal to 0.56 for Cr (VI) and 2.80 for Fe (III), and adsorption capacities are 18.15 mg/g and 39.80 mg/g, respectively. For activated carbon, depending on the raw materials [Table 2], 1/n varies from 0.181 for Pb (II) to 0.592 for Cr (VI) and adsorption capacities vary from 37.14 mg/g for Cd (II) to 253.2 mg/g for Pb (II). The characteristics of heterocyclic linear polymers are compared to those of the conventional adsorbents to select the best of them. Biosourced PIs[99] present a value of 1/n equal to 0.064 for Ni (II) and PASs[110] has a value of 0.059 for Pb (II). Such low values were not obtained with conventional adsorbents. Moreover, certain heterocyclic linear polymers demonstrated selectivity for specific heavy metal ions; for example, poly(etherphosphine)[121] and diethylphosphonate-containing poly(arylene ether nitrile)[122] were selective for Pb(II). This selectivity is particularly relevant for the recovery of pure heavy metals in diluted acidic media or complexing media. Concerning the adsorption capacities of heterocyclic linear polymers for Pb (II), they could vary from less than 1 mg/g[121] to 403.1 mg/g[105] and 452.1 mg/g[104] with respectively a pyridine-containing polyamide and a poly(azomethine amide)s. To enhance the adsorption capacities of linear polymeric phases, inorganic compounds such as silica gel, graphene, graphene oxide (GO), clay, and monoliths can be coated with them. The resulting materials significantly present a larger specific surface area and then a higher adsorption capacity; an example shows that the capacity of adsorption of PI for Cu2+ ion increased by a factor of 77 after PI/silica coprecipitation[123]. Another way to increase the specific surface area is the electrospinning of the heterocyclic linear polymers. Amino-rich functionalized PIs-polyethyleneimine (PEI) fibers with a diameter of 13 µm and a surface roughness of Rq = 17.10 nm were successfully synthesized. These fibers present numerous amine groups at the surface and were able to adsorb Cr (VI) ions with a capacity of adsorption of 50 mg/g[124]. Electrospun Fe3O4/MWCNTs/polyamide 6 nanofibers with a diameter of 101 nm enabled the adsorption of Pb (II) ion through the OH groups at their surface; an adsorption capacity of 49.3 mg/g was obtained[125]. Electrospun PA6/CaO nanofibers presented an adsorption capacity of 39 mg/g for Pb (II) ions and 33 mg/g for Cr (VI) ions[126].
Adsorbent regeneration is important for repeated use in different applications, as well as for reducing the quantity of adsorbent material, which in turn lowers the cost of the adsorbent. Studies have shown that adsorbents based on aromatic linear polymers can be reused for numerous cycles without significant loss of adsorption capacity.
Pyridine-based polyamide designed for removing Pb(II) and Cu(II) ions was successfully regenerated with various acids, including H2SO4, HCl, and CH3COOH, and used for seven adsorption-desorption cycles
Figure 6. Adsorption-desorption of Pb2+ (A) and Cu2+ (B) onto adsorbent polyamide. From[105] with permission.
Similarly, polyamide and polythioamide bearing chlorobenzylidine moieties retain their original removal capacity for removing Cr2+, Pb2+, Cd3+, and Cu2+ after four consecutive adsorption-desorption cycles[103]. Adsorbent regeneration was carried out using an aqueous solution of HCl (2N). The reusability results, presented in Table 5, revealed that the capacities of both polyamide P3 and polythioamide P5 remained practically unchanged for all metals, even after four cycles of adsorption-desorption.
Metal ion adsorption capacity of polyamide P3 and polythioamide P5 after four cycles of adsorption-desorption[103]
Cycle no. | Adsorption capacity (mg/g) of polyamide P3 | |||
Pb(II) | Cu(II) | Cr(II) | Cd(III) | |
1 | 108.7 | 102.5 | 91.8 | 60.1 |
2 | 106.4 | 102.4 | 71.1 | 58.2 |
3 | 104.9 | 100.9 | 65.7 | 53.8 |
4 | 99.7 | 98.1 | 64.7 | 49.6 |
Adsorption capacity (mg/g) of polythioamide P5 | ||||
1 | 109.4 | 108.5 | 82.6 | 60.1 |
2 | 107.5 | 100.9 | 71.8 | 56.5 |
3 | 105.9 | 99.5 | 71.1 | 44.6 |
4 | 103.8 | 96.7 | 65.7 | 37.8 |
Bıyıkoğlu et al. also examined the reusability of poly(2-aminothiazole) P-2AT after four adsorption-desorption cycles[116]. To remove the previously adsorbed Ag+ ion, the P-2AT was treated with 0.1 M HNO3 solution. As shown in Figure 7, the Ag+ adsorption capacity was almost the same on the second use and decreased on the fourth cycle to 23.84 mg/g. This suggests that P-2AT can be used repeatedly to remove Ag (I) ions.
Figure 7. Adsorption capacity of Ag (I) onto poly(2-aminothiazole) after four adsorption cycles. From[116] with permission.
After selecting the best formula and configuration for the heterocyclic polymers, the further challenge is achieving large-scale production at a reduced cost.
CONCLUSION
This study aims to present an overview of heterocyclic polymer-based adsorbents for the extraction of heavy metals to reduce water pollution. The various parameters affecting the adsorption capacity of heavy metals are studied. The removal of heavy metals from wastewater through adsorption is a cost-effective alternative to conventional costly methods, making it an optimal choice for wastewater treatment. Heterocyclic linear polymers are effective adsorbents of toxic metals due to their chemical and physical stability, their specific affinity, their adsorption capacity that can be improved, and their easy regeneration. The heterocyclic functional groups contained in the polymer backbone are responsible for the increased adsorption efficiency and recyclability of heavy metal ions in aqueous media. These groups act as attractive chelating sites and tend to eliminate ions through electrostatic interactions. The regeneration of the adsorbent polymers showed that the adsorption-desorption process is reversible, indicating that aromatic linear polymers have a strong potential for reuse, as the active sites are physically bonded to the polymer backbone. The successful use of heterocyclic polymer-based materials also opens up new possibilities for eliminating hazardous organic pollution from wastewater.
DECLARATIONS
Authors’ contributions
Writing - original draft preparation: Brirmi NEH, Chabbah T, Chatti S, Marestin C
Conceptualization: Alimi K, Ben Romdhame H, Mercier R
Writing - review and editing: Jaffrezic-Renault N
Availability of data and materials
Not applicable.
Financial support and sponsorship
The authors would like to acknowledge the financial support of the POLYAM project, funded by the High Ministry of Education and Research in Tunisia. The CNRS is acknowledged for its support of the IRP NARES. The research was funded by the European Union Horizon 2020 (TUNTWIN) research and innovation program under grant agreement (No. 952306). The authors acknowledge the financial support from the EU H2020 WIDESPREAD Program entitled Bionanosens grant agreement (No. 951887).
Conflicts of interest
Jaffrezic-Renault N is an Editorial Board member of the journal Water Emerging Contaminants & Nanoplastics, while the other authors declared that there are no conflicts of interest.
Ethical approval and consent to participate
Not applicable.
Consent for publication
Not applicable.
Copyright
© The Author(s) 2024.
REFERENCES
1. Beidokhti MZ, Naeeni ST, Abdi Ghahroudi MS. Biosorption of nickel (II) from aqueous solutions onto pistachio hull waste as a low-cost biosorbent. Civ Eng J 2019;5:447-57.
2. Liu J, Liu YJ, Liu Y, Liu Z, Zhang AN. Quantitative contributions of the major sources of heavy metals in soils to ecosystem and human health risks: a case study of Yulin, China. Ecotoxicol Environ Saf 2018;164:261-9.
3. Shen F, Mao L, Sun R, Du J, Tan Z, Ding M. Contamination evaluation and source identification of heavy metals in the sediments from the Lishui River watershed, Southern China. Int J Environ Res Public Health 2019;16:336.
4. Sharma R, Saini KC, Rajput S, et al. Environmental friendly technologies for remediation of toxic heavy metals: pragmatic approaches for environmental management. In: Aravind J, Kamaraj M, Karthikeyan S, editors. Strategies and tools for pollutant mitigation. Cham: Springer International Publishing; 2022. pp. 199-223.
5. Malik L A, Bashir A, Qureashi A, Pandith A H. Detection and removal of heavy metal ions: a review. Environ Chem Lett 2019;17:1495-521.
6. Ishaq S, Jabeen G, Arshad M, et al. Heavy metal toxicity arising from the industrial effluents repercussions on oxidative stress, liver enzymes and antioxidant activity in brain homogenates of Oreochromis niloticus. Sci Rep 2023;13:19936.
7. Gutwiński P, Cema G, Ziembińska-buczyńska A, Wyszyńska K, Surmacz-górska J. Long-term effect of heavy metals Cr(III), Zn(II), Cd(II), Cu(II), Ni(II), Pb(II) on the anammox process performance. J Water Process Eng 2021;39:101668.
8. Özdemir S, Serkan Yalçın M, Kılınç E. Preconcentrations of Ni(II) and Pb(II) from water and food samples by solid-phase extraction using Pleurotus ostreatus immobilized iron oxide nanoparticles. Food Chem 2021;336:127675.
9. Ali S, Hussain S, Khan R, et al. Renal toxicity of heavy metals (cadmium and mercury) and their amelioration with ascorbic acid in rabbits. Environ Sci Pollut Res Int 2019;26:3909-20.
10. Byber K, Lison D, Verougstraete V, Dressel H, Hotz P. Cadmium or cadmium compounds and chronic kidney disease in workers and the general population: a systematic review. Crit Rev Toxicol 2016;46:191-240.
11. Liu S, Shi J, Wang J, Dai Y, Li H, Li J, Zhang P. Interactions between microplastics and heavy metals in aquatic environments: a review. Front Microbiol 2021;12:652520.
12. Duan C, Ma T, Wang J, Zhou Y. Removal of heavy metals from aqueous solution using carbon-based adsorbents: a review. J Water Process Eng 2020;37:101339.
13. Genchi G, Carocci A, Lauria G, Sinicropi MS, Catalano A. Nickel: human health and environmental toxicology. Int J Environ Res Public Health 2020;17:679.
14. Mokhtari N, Dinari M, Rahmanian O. Novel porous organic triazine-based polyimide with high nitrogen levels for highly efficient removal of Ni(II) from aqueous solution. Polym Int 2019;68:1178-85.
15. Patil R, Sontakke T, Biradar A, Nalage D. Zinc: an essential trace element for human health and beyond. Food Health 2023;5:13.
16. Kambe T, Taylor KM, Fu D. Zinc transporters and their functional integration in mammalian cells. J Biol Chem 2021;296:100320.
17. Taiwo AM, Oyeleye OF, Majekodunmi BJ, et al. Evaluating the health risk of metals (Zn, Cr, Cd, Ni, Pb) in staple foods from Lagos and Ogun States, Southwestern Nigeria. Environ Monit Assess 2019;191:167.
18. Shabbir Z, Sardar A, Shabbir A, et al. Copper uptake, essentiality, toxicity, detoxification and risk assessment in soil-plant environment. Chemosphere 2020;259:127436.
19. Leyssens L, Vinck B, Van Der Straeten C, Wuyts F, Maes L. Cobalt toxicity in humans-a review of the potential sources and systemic health effects. Toxicology 2017;387:43-56.
20. Fei Y, Hu YH. Design, synthesis, and performance of adsorbents for heavy metal removal from wastewater: a review. J Mater Chem A 2022;10:1047-85.
21. Esalah J, Husein MM. Removal of heavy metals from aqueous solutions by precipitation-filtration using novel organo-phosphorus ligands. Separation Sci Technol 2008;43:3461-75.
22. Dabrowski A, Hubicki Z, Podkościelny P, Robens E. Selective removal of the heavy metal ions from waters and industrial wastewaters by ion-exchange method. Chemosphere 2004;56:91-106.
23. Oatley-radcliffe DL, Walters M, Ainscough TJ, Williams PM, Mohammad AW, Hilal N. Nanofiltration membranes and processes: a review of research trends over the past decade. J Water Process Eng 2017;19:164-71.
24. Tran T, Leu H, Chiu K, Lin C. Electrochemical treatment of heavy metal-containing wastewater with the removal of COD and heavy metal ions: electrochemical treatment of heavy metal containing wastewater. J Chin Chem Soc 2017;64:493-502.
25. Aarfane A, Bensemlali M, Elmelouky A, et al. Electrocoagulation efficiency probed using electrochemical impedance spectroscopy. Chin J Chem Eng 2024;75:266-73.
26. Elgarahy A, Elwakeel K, Mohammad S, Elshoubaky G. A critical review of biosorption of dyes, heavy metals and metalloids from wastewater as an efficient and green process. Clean Eng Technol 2021;4:100209.
27. Masoumi A, Ghaemy M. Adsorption of heavy metal ions and azo dyes by crosslinked nanochelating resins based on poly(methylmethacrylate-co-maleic anhydride). Express Polym Lett 2014;8:187-96.
28. Chai WS, Cheun JY, Kumar PS, et al. A review on conventional and novel materials towards heavy metal adsorption in wastewater treatment application. J Clean Prod 2021;296:126589.
29. Samiey B, Cheng CH, Wu J. Organic-inorganic hybrid polymers as adsorbents for removal of heavy metal ions from solutions: a review. Materials 2014;7:673-726.
30. Geng Z, Lin Y, Yu X, et al. Highly efficient dye adsorption and removal: a functional hybrid of reduced graphene oxide-Fe3O4 nanoparticles as an easily regenerative adsorbent. J Mater Chem 2012;22:3527.
31. Crini G. Recent developments in polysaccharide-based materials used as adsorbents in wastewater treatment. Prog Polym Sci 2005;30:38-70.
32. Chakraborty R, Asthana A, Singh AK, Jain B, Susan ABH. Adsorption of heavy metal ions by various low-cost adsorbents: a review. Int J Environ Anal Chem 2022;102:342-79.
33. Oladoye PO. Natural, low-cost adsorbents for toxic Pb(II) ion sequestration from (waste)water: a state-of-the-art review. Chemosphere 2022;287:132130.
34. Abdollahi N, Moussavi G, Giannakis S. A review of heavy metals’ removal from aqueous matrices by metal-organic frameworks (MOFs): state-of-the art and recent advances. J Environ Chem Eng 2022;10:107394.
35. Sikiru S, Abiodun OA, Sanusi YK, et al. A comprehensive review on nanotechnology application in wastewater treatment a case study of metal-based using green synthesis. J. Environ Chem Eng 2022;10:108065.
36. Boraah N, Chakma S, Kaushal P. Attributes of wood biochar as an efficient adsorbent for remediating heavy metals and emerging contaminants from water: a critical review and bibliometric analysis. J Environ Chem Eng 2022;10:107825.
37. Abdel-magied AF, Abdelhamid HN, Ashour RM, et al. Magnetic metal-organic frameworks for efficient removal of cadmium(II), and lead(II) from aqueous solution. J Environ Chem Eng 2022;10:107467.
38. Gomri M, Abderrazak H, Chabbah T, et al. Adsorption characteristics of aromatic pollutants and their halogenated derivatives on bio-based poly (ether-pyridine)s. J Environ Chem Eng 2020;8:104333.
39. Brirmi NEH, Chabbah T, Chatti S, et al. Effect of the pendent groups on biobased polymers, obtained from click chemistry suitable, for the adsorption of organic pollutants from water. Polym Adv Technol 2022;33:3551-71.
40. Gupta S, Sireesha S, Sreedhar I, Patel C M, Anitha K L. Latest trends in heavy metal removal from wastewater by biochar based sorbents. J Water Process Eng 2020;38:101561.
42. Ali H, Khan E. Bioaccumulation of non-essential hazardous heavy metals and metalloids in freshwater fish. Risk to human health. Environ Chem Lett 2018;16:903-17.
43. Paithankar JG, Saini S, Dwivedi S, Sharma A, Chowdhuri DK. Heavy metal associated health hazards: an interplay of oxidative stress and signal transduction. Chemosphere 2021;262:128350.
44. Rae IB, Gibb SW, Lu S. Biosorption of Hg from aqueous solutions by crab carapace. J Hazard Mater 2009;164:1601-4.
45. Hu XF, Lowe M, Chan HM. Mercury exposure, cardiovascular disease, and mortality: a systematic review and dose-response meta-analysis. Environ Res 2021;193:110538.
46. Chen R, Xu Y, Xu C, et al. Associations between mercury exposure and the risk of nonalcoholic fatty liver disease (NAFLD) in US adolescents. Environ Sci Pollut Res Int 2019;26:31384-91.
47. Hensawang S, Chanpiwat P. Health impact assessment of arsenic and cadmium intake via rice consumption in Bangkok, Thailand. Environ Monit Assess 2017;189:599.
48. Genchi G, Sinicropi MS, Lauria G, Carocci A, Catalano A. The effects of cadmium toxicity. Int J Environ Res Public Health 2020;17:3782.
49. Musfirah M, Rangkuti AF. The lead exposure risk due to wells water consumption in code riverside community, Yogyakarta city. Kemas 2019;14:318-25.
50. Thongsringklee M, Robson MG, Siriwong W. Health effects of low level exposure to lead among communication radio repair workers at Samutsakhon province, Thailand. Hum Ecol Risk Assess 2021;27:344-51.
51. Rizwan M, Ali S, Hussain A, et al. Effect of zinc-lysine on growth, yield and cadmium uptake in wheat (Triticum aestivum L.) and health risk assessment. Chemosphere 2017;187:35-42.
52. Yahya EB, Alqadhi AM. Recent trends in cancer therapy: a review on the current state of gene delivery. Life Sci 2021;269:119087.
53. Ren C, Zhou Y, Liu W, Wang Q. Paradoxical effects of arsenic in the lungs. Environ Health Prev Med 2021;26:80.
54. Rahaman MS, Rahman MM, Mise N, et al. Environmental arsenic exposure and its contribution to human diseases, toxicity mechanism and management. Environ Pollut 2021;289:117940.
55. Rahman Z, Singh V P. The relative impact of toxic heavy metals (THMs) (arsenic (As), cadmium (Cd), chromium (Cr)(VI), mercury (Hg), and lead (Pb)) on the total environment: an overview. Environ Monit Assess 2019;191:1-21.
56. Yoshinaga M, Ninomiya H, Al Hossain MMA, et al. A comprehensive study including monitoring, assessment of health effects and development of a remediation method for chromium pollution. Chemosphere 2018;201:667-75.
57. Peixoto S, Henriques I, Loureiro S. Long-term effects of Cu(OH)2 nanopesticide exposure on soil microbial communities. Environ Pollut 2021;269:116113.
58. Neff E, Dharmarajan G. The direct and indirect effects of copper on vector-borne disease dynamics. Environ Pollut 2021;269:116213.
59. Salih HHM, El Badawy AM, Tolaymat TM, Patterson CL. Removal of stabilized silver nanoparticles from surface water by conventional treatment processes. Adv Nanopart 2019;8:21-35.
60. Krou NJ. Etude expérimentale et modélisation d’un procédé séquentiel AD-OX d’élimination de polluants organiques. Génie des procédés 2010. (in French). Available from: https://theses.hal.science/tel-04272164. [Last accessed on 20 Nov 2024].
61. Taamneh Y, Sharadqah S. The removal of heavy metals from aqueous solution using natural Jordanian zeolite. Appl Water Sci 2017;7:2021-8.
62. Ugwu EI, Othmani A, Nnaji CC. A review on zeolites as cost-effective adsorbents for removal of heavy metals from aqueous environment. Int J Environ Sci Technol 2022;19:8061-84.
63. Taffarel SR, Rubio J. On the removal of Mn2+ ions by adsorption onto natural and activated Chilean zeolites. Min Eng 2009;22:336-43.
64. Álvarez AM, Guerrón DB, Montero Calderón C. Natural zeolite as a chromium VI removal agent in tannery effluents. Heliyon 2021;7:e07974.
65. Mehdi B, Belkacemi H, Brahmi-ingrachen D, Braham LA, Muhr L. Study of nickel adsorption on NaCl-modified natural zeolite using response surface methodology and kinetics modeling. Groundwater Sustain Dev 2022;17:100757.
66. Khalfa L, Sdiri A, Bagane M, Cervera ML. Multi-element modeling of heavy metals competitive removal from aqueous solution by raw and activated clay from the Aleg formation (Southern Tunisia). Int J Environ Sci Technol 2020;17:2123-40.
67. Dim PE, Termtanun M. Treated clay mineral as adsorbent for the removal of heavy metals from aqueous solution. Appl Sci Eng Prog 2021;14:511-24.
68. Angin D, Sarikulce S. The effect of activation temperature on properties of activated carbon prepared from wine industry pressing waste. Desal Water Treat 2017;73:373-9.
69. Zhang Z, Wang T, Zhang H, Liu Y, Xing B. Adsorption of Pb(II) and Cd(II) by magnetic activated carbon and its mechanism. Sci Total Environ 2021;757:143910.
70. Ghorbani F, Kamari S, Zamani S, Akbari S, Salehi M. Optimization and modeling of aqueous Cr(VI) adsorption onto activated carbon prepared from sugar beet bagasse agricultural waste by application of response surface methodology. Surf Interfaces 2020;18:100444.
71. Deng Y, Huang S, Laird DA, Wang X, Meng Z. Adsorption behaviour and mechanisms of cadmium and nickel on rice straw biochars in single- and binary-metal systems. Chemosphere 2019;218:308-18.
72. Lee ME, Park JH, Chung JW. Comparison of the lead and copper adsorption capacities of plant source materials and their biochars. J Environ Manage 2019;236:118-24.
73. Zhao W, Geng X, Lu J, et al. Mercury removal performance of brominated biomass activated carbon injection in simulated and coal-fired flue gas. Fuel 2021;285:119131.
74. Park JH, Wang JJ, Kim SH, et al. Cadmium adsorption characteristics of biochars derived using various pine tree residues and pyrolysis temperatures. J Colloid Interface Sci 2019;553:298-307.
75. Lata S, Singh PK, Samadder SR. Regeneration of adsorbents and recovery of heavy metals: a review. Int J Environ Sci Technol 2015;12:1461-78.
76. Afolabi F, Musonge P, Bakare B. Evaluation of lead (II) removal from wastewater using banana peels: optimization study. Pol J Environ Stud 2021;30:1487-96.
77. Çelebi H, Gök G, Gök O. Adsorption capability of brewed tea waste in waters containing toxic lead(II), cadmium (II), nickel (II), and zinc(II) heavy metal ions. Sci Rep 2020;10:17570.
78. Khalil U, Shakoor MB, Ali S, et al. Selective removal of hexavalent chromium from wastewater by rice husk: kinetic, isotherm and spectroscopic investigation. Water 2021;13:263.
79. Amar MB, Walha K, Salvadó V. Evaluation of olive stones for Cd(II), Cu(II), Pb(II) and Cr(VI) biosorption from aqueous solution: equilibrium and kinetics. Int J Environ Res 2020;14:193-204.
80. Varghese AG, Paul SA, Latha MS. Remediation of heavy metals and dyes from wastewater using cellulose-based adsorbents. Environ Chem Lett 2019;17:867-77.
81. Begum S, Yuhana NY, Md Saleh N, Kamarudin NHN, Sulong AB. Review of chitosan composite as a heavy metal adsorbent: material preparation and properties. Carbohydr Polym 2021;259:117613.
82. Tarabukin DV, Torlopov MA, Shchemelinina TN, et al. Biosorbents based on esterified starch carrying immobilized oil-degrading microorganisms. J Biotechnol 2017;260:31-7.
83. Yu D, Wang Y, Wu M, Zhang L, Wang L, Ni H. Surface functionalization of cellulose with hyperbranched polyamide for efficient adsorption of organic dyes and heavy metals. J Clean Prod 2019;232:774-83.
84. Guleria A, Kumari G, Lima EC. Cellulose-g-poly-(acrylamide-co-acrylic acid) polymeric bioadsorbent for the removal of toxic inorganic pollutants from wastewaters. Carbohydr Polym 2020;228:115396.
85. Yin W, Liu L, Tang S, Zhang H, Pan X, Chi R. Facile synthesis of triazole and carboxyl-functionalized cellulose-based adsorbent via click chemistry strategy for efficient Gd(III) removal. Cellulose 2019;26:7107-23.
86. Mahalakshmi R, Saravanan R, Selvakumar P, Karthikeyan MS, Ravikumar L. Polymeric substitution of triazole moieties in cellulosic schiff base for heavy metal complexation studies. J Polym Environ 2022;30:360-72.
87. Dinari M, Mokhtari N, Hatami M. Covalent triazine based polymer with high nitrogen levels for removal of copper (II) ions from aqueous solutions. J Polym Res 2021;28:2463.
88. Chu S, Feng X, Liu C, Wu H, Liu X. Advances in chelating resins for adsorption of heavy metal ions. Ind Eng Chem Res 2022;61:11309-28.
89. Wu H, Lin G, Liu C, Chu S, Mo C, Liu X. Progress and challenges in molecularly imprinted polymers for adsorption of heavy metal ions from wastewater. Trends Environ Anal Chem 2022;36:e00178.
90. Bazzar M, Ghaemy M, Alizadeh R. Synthesis and characterization of new fluorescent polyimides bearing 1,2,4-triazole and 1,2-diaryl quinoxaline: study properties and application to the extraction/elimination of metallic ions from aqueous media. React Funct Polym 2013;73:492-8.
91. Ghaemy M, Qasemi S, Ghassemi K, Bazzar M. Nanostructured composites of poly(triazole-amide-imide)s and reactive titanium oxide by epoxide functionalization: thermal, mechanical, photophysical and metal ions adsorption properties. J Polym Res 2013;20:278.
92. Ghaemy M, Ghassemi K, Qasemi S, Bazzar M. Synthesis, characterization and properties of new poly(triazole-imide)s and their composites with silane-modified nanoclay. Polym Bull 2015;72:2435-53.
93. Amininasab SM, Esmaili S, Taghavi M, Shami Z. Fabrication and characterization of novel high-performance fluorinated polyimides with xanthene pendent architecture: study of thermal, photophysical, antibacterial and heavy metal ion adsorption behavior. React Funct Polym 2016;192:48-57.
94. Amininasab SM, Esmaili S, Shami Z. High-performance polyimides based on pyridine and xanthene pendant groups; synthesis, characterization, photoactivity, thermal, antibacterial, and Cr(VI) ion adsorption properties. High Perform Polym 2020;32:371-82.
95. Huang X, Li H, Liu C, Wei C. Design and synthesis of high heat-resistant, soluble, and hydrophobic fluorinated polyimides containing pyridine and trifluoromethylthiophenyl units. High Perform Polym 2019;31:107-115.
96. Ghorbani M, Eisazadeh H. Removal of COD, color, anions and heavy metals from cotton textile wastewater by using polyaniline and polypyrrole nanocomposites coated on rice husk ash. Compos Part B Eng 2013;45:1-7.
97. Rafiee Z, Golriz L, Karimipour G, Kowkabi S. Preparation and properties of polyimides having porphyrin moieties for heavy metals removal. Polym Bull 2021;78:481-92.
98. Natale C, Monti D, Paolesse R. Chemical sensitivity of porphyrin assemblies. Mater Today 2010;13:46-52.
99. Jlalia I, Zouaoui F, Chabbah T, et al. Adsorption characteristics of WFD heavy metal ions on new biosourced polyimide films determined by electrochemical impedance spectroscopy. J Inorg Organomet Polym 2021;31:2471-82.
100. Lakouraj MM, Rahpaima G, Azimi R. Organosoluble xanthone-based polyimides: synthesis, characterization, antioxidant activity and heavy-metal sorption. Mater Tehnol 2016;50:471-8.
101. Mansoori Y, Ghanbari M. Novel polyimides obtained from a new aromatic diamine (BAPO) containing pyridine and 1,3,4-oxadiazole moieties for removal of Co(II) and Ni(II) ions. Polymers for Advanced Techs 2015;26:658-64.
102. Manzoor A, Kalsoom S, Khan YK, Siddiqi HM, Shah MH. Synthesis of sulfonated copolyimides by thermal imidization for efficient lead ion adsorption from aqueous media. ACS Appl Polym Mater 2022;4:5660-9.
103. Ravikumar L, Kalaivani SS, Murugesan A, et al. Synthesis, characterization, and heavy metal ion adsorption studies of polyamides, polythioamides having pendent chlorobenzylidine rings. J of Applied Polymer Sci 2011;122:1634-42.
104. Murugesan A, Ravikumar L, Sathyaselvabala V, et al. Removal of Pb(II), Cu(II) and Cd(II) ions from aqueous solution using polyazomethineamides: Equilibrium and kinetic approach. Desalination 2011;271:199-208.
105. Kirupha SD, Murugesan A, Vidhyadevi T, Baskaralingam P, Sivanesan S, Ravikumar L. Novel polymeric adsorbents bearing amide, pyridyl, azomethine and thiourea binding sites for the removal of Cu(II) and Pb(II) ions from aqueous solution. Separation Sci Technol 2012;48:254-62.
106. Gómez-valdemoro A, San-josé N, García FC, De La Peña JL, Serna F, García JM. Novel aromatic polyamides with main chain and pendant 1,2,4-triazole moieties and their application to the extraction/elimination of mercury cations from aqueous media. Polym Chem 2010;1:1291.
107. Soleimani B, Taghavi M, Ghaemy M. Synthesis, characterization and heavy metal ion adsorption behavior of imidazole-based novel polyamides and polyimides. J Mex Chem Soc 2017:61.
108. Santiago AA, Ibarra-palos A, Cruz-morales JA, et al. Synthesis, characterization, and heavy metal adsorption properties of sulfonated aromatic polyamides. High Perform Polym 2018;30:591-601.
109. Albukhari SM, Hussein MA, Abdel Rahman MA, Marwani HM. Highly selective heteroaromatic sulfur containing polyamides for Hg+2 environmental remediation. Des Monomers Polym 2020;23:25-39.
110. Rezania H, Vatanpour V, Salehi E, Gavari N, Shockravi A, Ehsani M. Wholly heterocycles-based polyamide-sulfide containing pyridine and thiazole rings: a super-adsorbent polymer for lead removal. J Polym Environ 2019;27:1790-800.
111. Hsiao S, Guo W, Kung Y, Lee Y. Redox-active and electrochromic aromatic poly(amide-imide)s with 2,4-dimethoxytriphenylamine chromophores. J Polym Res 2011;18:1353-64.
112. Faghihi K, Hajibeygi M, Shabanian M. New photosensitive and optically active organo-soluble poly(amide-imide)s from N,N′-(bicyclo[2,2,2]oct-7-ene-tetracarboxylic)-bis-L-amino acids and 1,5-bis(4-aminophenyl)penta-1,4-dien-3-one: synthesis and characterization. J Polym Res 2010;17:379-90.
113. Thiruvasagam P, Vijayan M. Synthesis of new diacid monomers and poly(amide-imide)s: study of structure-property relationship and applications. J Polym Res 2012;19:9845.
114. Alborzi A, Zahmatkesh S, Yazdanpanah A. l-Lysine-derived optically active poly(hydrazide-imide)s: synthesis, characterization and their application in removal of heavy metal ions. Polym Bull 2013;70:3359-72.
115. Vakili MR, Zahmatkesh S, Panahiyan MJ, Jafarizadeh T. Poly(amide-hydrazide-imide)s containing L-aspartic acid: synthesis, characterization, and their applications in removal of heavy metal ions. Des Monomers Polym 2015;18:315-22.
116. Bıyıkoğlu M, Çiftçi H. Adsorption of Ag(I) ions from wastewaters using poly(2-aminothiazole): kinetic and isotherm studies. Polym Bull 2020;77:6161-74.
117. Puguan JMC, Kim H. Synthesis of free-standing poly(ionic liquid) bearing 1,2,3-triazole group for the adsorptive elimination of Cr6+ from aqueous solution. J Environ Chem Eng 2020;8:104084.
118. Ouerghui A, Dardouri M, Elamari H, Ammari F, Girard C. Chemical modification of polystyrene merrifield: extraction of zinc and magnesium located in wastewater. AJPST 2019;5:73.
119. Qureshi F, Memon SQ, Khuhawar MY, Jahangir TM. Removal of Co2+, Cu2+ and Au3+ ions from contaminated wastewater by using new fluorescent and antibacterial polymer as sorbent. Polym Bull 2021;78:1505-33.
120. Qureshi F, Memon SQ, Khuhawar MY, Jahangir TM, Channar A. Synthesis and application of fluorescent and thermally stable polyazomethine as adsorbent in the remediation of Ni (II), Cu (II) and Co (II) from wastewater systems. J Polym Res 2021;28:2582.
121. Chabbah T, Abderrazak H, Souissi R, et al. A sensitive impedimetric sensor based on biosourced polyphosphine films for the detection of lead ions. Chemosensors 2020;8:34.
122. Chabbah T, Chatti S, Jaffrezic-renault N, Weidner S, Marestin C, Mercier R. Impedimetric sensors based on diethylphosphonate-containing poly(arylene ether nitrile)s films for the detection of lead ions. Polym Adv Technol 2023;34:2471-81.
123. Huang J, Zheng Y, Luo L, et al. Facile preparation of highly hydrophilic, recyclable high-performance polyimide adsorbents for the removal of heavy metal ions. J Hazard Mater 2016;306:210-9.
124. Jia W, Du J, Jiang M, et al. Preparation and Cr (VI) adsorption of functionalized polyimide fibers. J of Applied Polymer Sci 2022;139:e52799.
125. Bassyouni D, Mohamed M, El-ashtoukhy E, El-latif MA, Zaatout A, Hamad H. Fabrication and characterization of electrospun Fe3O4/o-MWCNTs/polyamide 6 hybrid nanofibrous membrane composite as an efficient and recoverable adsorbent for removal of Pb (II). Microchem J 2019;149:103998.
Cite This Article
How to Cite
Brirmi, N. E. H.; Chabbah, T.; Chatti, S.; Alimi, K.; Romdhame, H. B.; Mercier, R.; Marestin, C.; Jaffrezic-Renault, N. Progress and challenges in heterocyclic polymers for the removal of heavy metals from wastewater: a review. Water Emerg. Contam. Nanoplastics. 2024, 3, 23. http://dx.doi.org/10.20517/wecn.2024.60
Download Citation
Export Citation File:
Type of Import
Tips on Downloading Citation
Citation Manager File Format
Type of Import
Direct Import: When the Direct Import option is selected (the default state), a dialogue box will give you the option to Save or Open the downloaded citation data. Choosing Open will either launch your citation manager or give you a choice of applications with which to use the metadata. The Save option saves the file locally for later use.
Indirect Import: When the Indirect Import option is selected, the metadata is displayed and may be copied and pasted as needed.
About This Article
Copyright
Data & Comments
Data
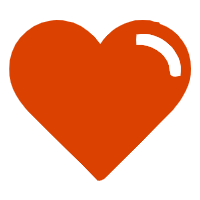
Comments
Comments must be written in English. Spam, offensive content, impersonation, and private information will not be permitted. If any comment is reported and identified as inappropriate content by OAE staff, the comment will be removed without notice. If you have any queries or need any help, please contact us at support@oaepublish.com.