Utilizing microorganisms immobilized on carbon-based materials for environmental remediation: a mini review
Abstract
Carbon-based materials have attracted significant interest due to their porous structure and high specific surface area. The stability of carbon provides an optimal environment for microbial growth and activity. This paper presents a comprehensive review of microorganisms immobilized on carbon-based materials. Techniques used to immobilize microorganisms on various carbon-based materials are discussed initially. Subsequently, the review introduces the application of these immobilized microorganisms in the restoration of soil and water environments, including the treatment of organic pollutants, heavy metals, and ammonia nitrogen. Overall, this work enhances our understanding of the microbial pathways facilitated by carbon-based materials.
Keywords
INTRODUCTION
Amid the rapid progression of urbanization and industrialization, toxic pollutants are continuously discharged into the environment, accumulating in the air, water, and soil, and ultimately infiltrating the human body, causing harm to human health. According to the World Health Organization (WHO), nearly a quarter of all human diseases today can be traced back to prolonged exposure to environmental pollution. This indicates the pressing need for effective strategies to mitigate environmental pollution and its impacts on human health[1]. Indeed, environmental pollution remains a major global challenge, and the implementation of appropriate environmental remediation technologies is crucial for preserving human health and safety. Currently, remediation strategies for environmental pollution are primarily classified into physical, chemical, and biological remediation technologies[2]. Among these, biological remediation methods have garnered increasing attention due to their cost-effectiveness and wide applicability. This underscores the potential of microbial remediation as a sustainable and efficient solution for environmental pollution. Nonetheless, although the microbial method for environmental remediation has its advantages, it also has certain limitations. These include the reduction or loss of microbial activity, challenges in separation and recovery post-adsorption, poor genetic stability of microbes leading to easy alterations, and limited adsorption capacity for heavy metals[3]. Consequently, current research is focused on effectively enhancing the treatment efficacy of the microbial method and improving microbial activity. This underscores the necessity for innovative solutions to overcome these limitations and unlock the full potential of microbial remediation for environmental pollution.
Microbial immobilization technology is indeed widely regarded as a potentially effective approach. The process of immobilizing microorganisms offers numerous advantages including high biomass, stability, resistance to loss, rapid reaction speed, strong anti-toxicity, and ease of separation[4]. However, the carrier material employed in this process can exhibit weak mechanical stability and may release toxic elements, leading to a loss of microbial activity. Therefore, the selection of the carrier material is a pivotal aspect of microbial immobilization technology. Despite the challenges that require further investigation, particularly in the selection of carrier materials, the ideal immobilized carrier material should possess attributes such as high biocompatibility, accessibility, stability, a large specific surface area, and low cost. This underscores the necessity for ongoing research in this area to optimize the effectiveness of microbial immobilization technology.
Currently, the primary materials used as carriers include polymers, metal nanoparticles, organometallic frameworks, and carbon materials[5]. Among these, carbon materials have emerged as an ideal platform for microbial carrier materials in recent years. This is attributed to their stable structure, abundant pore structure, and large specific surface area. Additionally, they exhibit excellent biocompatibility, low cost, and are rich in functional groups such as hydroxyl and carboxyl groups. These characteristics render carbon materials particularly suitable for microbial immobilization, enhancing the efficacy of this approach for environmental remediation[6]. However, further research is required to fully exploit the potential of these materials and address any associated challenges. As the use of carbon-based materials for microbial immobilization continues to rise, these materials are assuming an increasingly important role in the treatment of environmental pollutants [Figure 1].
Figure 1. The number of immobilized microorganisms in different materials published per year (Search keywords: immobilized microorganism, biochar, metal oxide, polymer, carbon-based materials. Source: Web of Science, search date: 06-30-2024).
In recent years, some researchers have scrutinized the potential of microbial immobilization techniques in various environmental remediation contexts, introducing a diversity of carrier materials and fixation methods, and deliberating on the factors influencing the microbial immobilization process[7,8]. However, despite the escalating utilization of carbon-based materials in microbial immobilization, a comprehensive review of the application of carbon-based immobilized microorganisms in the treatment of environmental pollutants remains absent.
To address this lacuna, we embarked on a meticulous examination of the literature spanning the past two decades, culminating in this exhaustive review. Consequently, we present an overview of the methodologies for immobilizing microorganisms using carbon-based materials such as biochar, activated carbon, carbon nanotubes, and graphene. We further explore their application in the removal of pollutants from aquatic and soil environments. This review aims to serve as a valuable reference for future research on the use of carbon-based materials for microbial immobilization in the remediation of water and soil pollution.
CARBON-BASED MATERIALS
The selection of the carrier material is a crucial factor influencing the immobilization of microorganisms. Different raw materials, along with varying preparation methods and processes, yield different material properties. Key characteristics to consider when selecting a carrier material include its specific surface area, pore size, structure, and mechanical strength, as these significantly impact the microbial load[9].
Recently, carbon-based materials have emerged as some of the most promising nanostructured materials. These materials have garnered significant attention due to their distinctive characteristics, which include high porosity, extensive surface area, functional groups, modifiable surfaces, and a predominantly aromatic structure. Therefore, they are frequently employed as versatile carriers for microbial immobilization. In addition, a thorough understanding of the different characteristics of these carbon-based materials is essential for the effective application of microbial immobilization technology in environmental remediation[10,11]. This highlights the need for continued research and development in this area to identify and optimize the use of suitable carrier materials.
Biochar
Biochar has gained significant attention in recent years as an effective immobilization matrix. Its high carbon content, cation exchange capacity, porosity, stability, and abundance of surface functional groups make it an ideal choice for microbial immobilization[12]. These properties allow biochar to support high enrichment of microorganisms, thereby enhancing the effectiveness of the microbial method for environmental remediation.
This underscores the potential of biochar as a sustainable and efficient solution for environmental pollution. However, further research is needed to fully exploit the potential of biochar and address associated challenges, such as low microbial activity and microbial enrichment and other problems. To address these issues, Han et al. investigated the loading of Bacillus subtilis 168 onto biochar modified with acid and quaternary ammonium salts to enhance the removal of zearalenone[13]. The study’s findings indicated that the modified biochar exhibited an improved pore structure (27.26 nm) compared to pristine biochar
To date, the challenge of recovering powdered biochar from aqueous solutions remains a significant limitation to its application. To address this, Zheng et al. developed an innovative embedding method by preparing biochar fungal pellets (BFP) that immobilized denitrifying bacteria (Cupriavidus sp. H29) for use in bioreactors[16]. The results revealed that the BFP, with its loose and abundant filamentous void structures and a particle size of 5 mm, not only provided ample space and favorable conditions for bacterial adhesion and reproduction but also exhibited excellent solid-liquid separation and reuse performance. Xiang et al. present an innovative approach to Chryseobacterium spH5 strains immobilization using a bead composed of polyvinyl alcohol (PVA), sodium alginate (SA), and biochar for thiamethoxam degradation[17]. The results revealed that the PVA/SA/biochar (0.7%) immobilized microorganisms demonstrated a superior removal effect on thiamethoxam, achieving a removal rate of 90.47%. This was significantly higher compared to the removal rates achieved by PVA/SA immobilized microorganisms (75.06%) and free microorganisms (61.72%). Furthermore, the PVA/SA/biochar (0.7%) immobilized microorganisms exhibited excellent tolerance under extreme environmental conditions. Zeng et al. provide valuable insights into the use of ferric tetroxide-modified biochar nanomaterials for the immobilization of Alcaligenes faecalis strains[18]. The results demonstrated that the bacteria could sustain growth and maintain robust activity within 48 h under the conditions of 25-40 °C, pH 5-9, initial phenol concentration of 300-500 mg/L, and salinity of 3%.
This demonstrated the potential of modified biochar as an efficacious carrier material for microbial immobilization in environmental remediation. However, further research is required to optimize these modifications and fully harness the potential of biochar.
Activated carbon
Activated carbon, with its large specific surface area and rich pore structure, is highly conducive to cell attachment, making it a popular choice as a solid carrier for the removal of environmental pollutants[19]. As early as 1991, Lin et al. explored the co-immobilization system of activated carbon and Phanerochaete chrysosporium for the degradation of pentachlorophenol (PCP)[20]. The findings revealed that the normalized PCP mineralization by the co-immobilization system reached 68%. In contrast, only about 17% of the normalized PCP mineralization was observed for the non-immobilized system at 120 h. This is attributed to the solid substrate-activated carbon present in the co-immobilization system, which enhances biodegradation and stability. Furthermore, Annadurai et al. demonstrated that the immobilization of microorganisms on activated carbon can notably enhance the degradation efficiency of pollutants, while simultaneously shielding the microorganisms from the impact load of organic pollutants[21]. Hence, the structural characteristics of activated carbon are crucial in microbial loading. Maharaja et al. developed a nanoporous activated carbon (NPAC) for the immobilization of microorganisms in bioreactors[22]. The findings indicate that the BET surface area of NPAC is 291 m2/g and the average pore diameter is 28 Å. The overall removal efficiency of chemical oxygen demand (COD) and biological oxygen demand (BOD) in the reactor are 82% and 85%, respectively, demonstrating the effective carrier properties of NPAC.
To further enhance the efficiency of pollutant removal, Yang et al. employed three different methods to modify coconut shell activated carbon: acid, water, and alkali[23]. This modified activated carbon was used to immobilize two microbial communities (ERY-6A and ERY-6B) aimed at degrading erythromycin. The results indicated that both alkali-modified and water-modified activated carbon, in conjunction with the two-bacteria system, exhibited effective erythromycin removal capabilities. The removal efficiency of erythromycin by immobilized cells reached 94.36% within 24 h, which is significantly higher than the 31.90% removal rate by free bacteria. Erythromycin was eliminated by the immobilized cells through mechanisms such as pore adsorption, surface complexation, hydrogen bonding, and biodegradation. Thus, activated carbon also shows potential as a material for microbial immobilization.
Carbon nanotubes
Given that immobilization can augment the activity and stability of microorganisms, carbon nanotubes (CNTs), serving as a carrier for microbial immobilization, have garnered increasing interest in the realm of environmental pollutant removal[24]. CNTs are nanoscale carbon-based materials with a unique surface structure. They offer distinctive properties for the immobilization of microorganisms, owing to their high surface area, superior thermodynamic properties, and low electroosmotic threshold. There exist several types of nanotubes, such as single-walled carbon nanotubes (SCNTs) and multi-walled carbon nanotubes (MCNTs)[25]. While SCNTs consist of only one central carbon tubule, MCNTs are composed of multiple layers of graphite encircling a central carbon tubule. In comparison to SCNTs, MCNTs possess a higher surface area, better dispersibility, and enhanced physicochemical stability, while being more cost-effective and exhibiting lower cytotoxicity[26].
Akyilmaz et al. immobilized Candida tropicalis onto carbon paste electrodes that contained SCNTs for the detection of dopamine and adrenaline[27]. In a separate study, Boshagh et al. examined the hydrogen production process of Enterobacter aerogenes PTCC 1221 immobilized by MWCNTs-COOH[28]. The results revealed that MWCNTs-COOH had a higher hydrogen production rate (2.72 L/L·h) than Enterobacter aerogenes PTCC 1221. Fourier transform infrared (FTIR) spectroscopy confirmed the successful covalent attachment of the aerogenes to MWCNT-COOH, primarily attributed to the formation of amide bonds between the carboxylic acid groups of the functionalized molecules and the amide groups of the aerogenes.
Therefore, Perchikov et al. immobilized cells in a redox-active polymer based on ferrocenecarboxaldehyde-modified bovine serum albumin and CNTs composite for the rapid detection of the phenol index[29]. The findings showed that the detection rate of the redox-active polymer containing CNTs was 502.8 dm3/(g·s), compared to 193.8 dm3/(g·s) for the redox-active polymer without carbon nanotubes. Therefore, CNTs-based composites show promise as a potential new material for microbial immobilization.
Graphene
Graphite, graphene and graphene derivative, graphene oxide (GO), is a representative carbon nanomaterial, notable for its large specific surface area and excellent thermal and chemical stability[30]. Despite its inherent antibacterial properties, GO can also foster the growth of microorganisms by adsorbing proteins and supplying ample nutrients, thereby neutralizing its antibacterial capabilities. Consequently, GO can serve as a carrier material for microbial immobilization. Zhao et al. constructed microbe- GO composites to adsorb uranium (VI) from aqueous solutions, achieved by immobilizing Lysinibacillus sp[31]. The results demonstrated that the maximum adsorption capacity of Lysinibacillus-GO for U (VI) was 149.3 mg/g. The primary mechanism of adsorption is attributed to the inner-sphere complexation between U (VI) and oxygen-containing groups.
Liu et al. demonstrate the potential of GO/SA aerogel as an adsorption material[32]. By immobilizing heterotrophic nitrifying bacteria FG-06 onto this aerogel, they were able to enhance both the growth of the bacteria and the removal of pollutants. This suggests that GO/SA/FG-06 could be more effective than traditional suspension agents in certain applications. It is another promising step toward more efficient and effective environmental cleanup strategies.
To further enhance environmental applicability, Yong et al. developed a method for the immobilization of electroactive microorganisms (Shewanella oneidensis MR-1) in microbial fuel cells (MFCs) using graphite/alginate particles[33]. The results indicated that the MFC output of the immobilized cell particles was more stable than that of the suspended cells, and the Coulombic efficiency improved by 0.8-1.7 times compared to the suspended mode. Additionally, MFCs with immobilized cells demonstrated greater resistance to shock from high salt concentrations than MFCs with suspended cells. In another study, Li et al. first co-deposited carboxyl graphene (GN-COOH) and gold nanoparticles (AuNPs) onto an electrode, and then immobilized the microbe (Bacillus subtilis) onto the modified electrode via covalent bonds[34]. The results showed that this approach shortened the distance between the microbe and the electrode, which is beneficial for electron and mass transfer. Therefore, nanocomposites based on graphene also present a promising new option for microbial immobilization. These studies highlight the potential of carbon-based materials in enhancing the efficiency and stability of microbial processes in environmental applications.
In conclusion, based on current research and technological advancements, biochar offers notable advantages in terms of cost and environmental benefits; however, its performance still requires further enhancement. Activated carbon excels in microbial immobilization due to its high specific surface area and effective adsorption capabilities, but issues related to cost and regeneration need to be addressed. Carbon nanotubes and graphene show significant promise for providing exceptional support and performance, yet challenges related to high costs and environmental impact persist. Future research should concentrate on reducing production costs, enhancing functionalization methods, and evaluating long-term environmental effects to fully harness the potential of these materials in microbial immobilization technologies.
THE FACTORS AFFECTING THE MICROBIAL IMMOBILIZATION
The immobilization of microorganisms on carbon-based materials is influenced by both the intrinsic properties of the carbon-based substrates and a spectrum of environmental factors, such as the initial pollutant concentration, ambient temperature, pH levels, and contact duration. These variables collectively influence the degradation efficiency of pollutants. Consequently, it is imperative to investigate the optimal immobilization conditions to enhance the application of microbial immobilization technology in pollutant removal.
The effect of initial pollutant concentration
Pollutant concentration is a critical parameter in the degradation pathway. Generally, increasing the initial concentration of contaminants enhances their adsorption onto the material until saturation is reached. However, elevated concentrations of pollutants can exert toxic effects on microorganisms, thereby inhibiting their activity. Thus, selecting appropriate carbon-based materials for immobilizing microbial carriers can shield microorganisms from pollutant-induced toxicity, thereby maximizing their pollutant degradation potential.
For instance, Xiang et al. observed that increasing the concentration of thiamethoxam from 7 to 42 mg/L resulted in a decrease in the degradation efficiency of free microorganisms from 77.30% to 45.70%[17]. Similarly, the removal efficiency of PVA/SA/biochar-immobilized microorganisms for thiamethoxam decreased from 94.94% to 80.13%. This demonstrates the efficacy of PVA/SA/biochar in protecting microorganisms under high concentrations of contaminants.
pH
pH is another critical parameter; variations in pH not only significantly impact the growth of microorganisms but also alter the surface characteristics of materials and the behavior of pollutants in solution, thereby playing a pivotal role in pollutant removal. Zhang et al. observed that biochar-immobilized LZ01 exhibited a higher removal rate of chlortetracycline in neutral and slightly alkaline environments, reaching up to 82.39%[35]. In contrast, the removal rate of chlortetracycline by free LZ01 was 10.31%. Consequently, the influence of pH on the removal rate of immobilized LZ01 is less pronounced compared to free LZ01, primarily because biochar protects microorganisms from adverse pH conditions, thereby enhancing their chlortetracycline removal efficiency. Yu et al. proposed considering both the optimal pH for microbial growth and the ideal pH for carrier-microbial binding[36]. This approach can maximize the synergistic benefits of the carrier-microorganism combination in contaminant removal.
Temperature
Temperature is a crucial factor influencing both substance reactions and microbial activity. Generally, the optimal temperature for bioremediation is 25-35 °C, which limits its application under extreme conditions. Consequently, microbial immobilization technology holds promise for enhancing bioremediation in harsh conditions. Manikandan et al. observed that the removal rate of Ni2+ by wood biochar-immobilized Pseudomonas stutzeri increased with rising temperatures[37]. This increase may be attributed to the expansion of pore structures at higher temperatures, creating more surface-active sites for metal ion adsorption. Additionally, temperature influences the solubility of metals and the membrane binding affinity of metal ions to the bacterial cell wall.
The effect of contact time
Contact time is a critical factor influencing the microbial growth curve and cellular activity levels. Zhang
ENVIRONMENTAL APPLICATIONS
The rapid growth of population, industry, urbanization, and intensive agriculture has led to the pollution of soil and water by various pollutants. Microbial immobilization technology has become one of the most successful emerging technologies for soil and water pollution remediation. Among them, Carbon-based materials that immobilize microorganisms have shown great promise in environmental remediation[38]. It has been successfully used to remove a variety of organic and inorganic pollutants from different mediums such as water, soil, and air. These pollutants primarily include toxic metals and organic contaminants[39]. The removal process typically involves biosorption and/or biodegradation mechanisms. This innovative approach leverages the natural abilities of microorganisms and the unique properties of carbon-based materials, offering a potentially effective and sustainable solution for pollution control.
Water pollution remediation
To date, the excessive presence of pollutants in water has detrimental effects on water quality, posing significant health risks[40]. Therefore, it is crucial to explore economical, scalable, efficient, and rapid methods for water treatment. Microbial immobilization methods are emerging as promising technologies for managing water quality. These methods leverage the natural abilities of microorganisms to detect, monitor, and remove target water contaminants. The use of carbon-based materials for microbial immobilization, as discussed earlier, can enhance the efficiency and stability of these processes[41]. These methods are not only cost-effective but also environmentally friendly, making them an attractive option for water treatment. They represent a significant advancement in the field of environmental science and hold great potential for addressing the pressing issue of water pollution. It is an exciting area of research with the potential to make a substantial impact on our environment and health. An et al. demonstrated the effectiveness of using Pseudomonas hibiscicola L1 strain (Strain L1) immobilized in peanut shell biochar (PBC) for the removal of various pollutants from mixed electroplating wastewater[42]. The pollutants included nickel (II), zinc (II), copper (II), chromium (VI), and ammonia nitrogen. The results showed that PBC not only enhanced the biological activity of Strain L1, but also increased the removal rate of pollutants compared to using PBC alone or free Strain L1. Specifically, the removal rates of the biocarbon-immobilized Strain L1 in mixed electroplating wastewater were as follows: copper (II) (94.92%) > zinc (II) (91.46%) > ammonia nitrogen (79.90%) > nickel (II) (77.02%) > chromium (VI) (34.40%). The removal mechanism proposed by the authors is illustrated in Figure 2A. The removal process primarily involves four mechanisms: (1) nitrate in the solution is utilized by Strain L1 as a nitrogen source for denitrification, forming intermediate nitrite; (2) Cr (VI) is reduced to Cr (III) through electron acceptance by PBC; (3) certain heavy metal ions are removed from the solution via ion exchange; and (4) other heavy metal ions are removed through adsorption processes, which include interactions with functional groups (-OH, -COOH,
Figure 2. (A) Removal mechanism of co-system; (B) Schematic diagram of the role of immobilized growth cells in the mechanism of Cd2+ biosorption; (C) The removal of erythromycin by immobilized cells in mineral salt medium and the potential mechanisms.
Nowadays, the rapid industrial and agricultural development in recent years has led to the discharge of large amounts of difficult-to-treat wastewater. Generally, the wastewater contains high concentrations of biodegradable organic pollutants, and even after treatment, it usually fails to meet discharge standards, leading to serious water pollution issues.
In response to this issue, Ouyang et al. successfully prepared SA and biochar immobilized GYB1 beads (SC-GYB1) for the biodegradation of 2,3’,4,4’,5-pentachlorobenzene (PCB 118)[44]. Their results showed that with 2.0% SA, 2.0% wet weight, and 1.5% biochar content, they could degrade 50.50% of PCB 118 within 5 days. Impressively, SC-GYB1 maintained considerable PCB degradation capacity even under 200 mg/L Cd2+ stress. In another study, Lu et al. prepared Triton X-100 by an adsorption method, adding the non-ionic surfactant Triton X-100 and using biochar as an immobilized carrier to promote biochar immobilized Pseudomonas aeruginosa material[45]. This was used for the removal of naphthalene, a refractory organic matter in water. The results showed that low concentrations of Triton X-100 significantly increased the removal rate of naphthalene by 20%-50%, compared with no addition of Triton X-100. This approach not only improved the adsorption capacity of biochar but also promoted the degradation activity of Pseudomonas aeruginosa. The authors hypothesize that the two primary removal mechanisms are the adsorption of naphthalene by the biochar itself and the degradation of naphthalene by bacteria immobilized on the biochar.
To enhance pollutant removal efficiency, Yang et al. prepared coconut shell activated carbon (NMC) loaded with dual microbial flora (ERY-6A and auriferous bacterium ERY-6B) for erythromycin degradation[23]. The results demonstrated that the immobilized bacteria could remove 95% of 100 mg/L erythromycin within
These studies underscore the potential of using carbon-based materials and microbial immobilization for effective wastewater treatment. It is a promising approach toward more sustainable and efficient environmental remediation strategies.
Soil remediation
To date, soil pollution is a significant global issue. Specifically, the leakage and discharge of fossil fuels during extraction, storage, transportation, processing, and use can lead to the accumulation of toxic and harmful substances in the environment[46]. This not only poses a serious threat to human health but also endangers the safety of entire ecosystems. Therefore, soil remediation is an urgent necessity.
Yin et al. demonstrate the effectiveness of using rice husk biochar (RHB) and SA as immobilized substrates for complex fungi to create an adsorption system (CFIRHB) and an encapsulation system (CFI-RHB/SA)[47]. The results indicated that CFI-RHB/SA had the highest diesel removal efficiency (64.10%) in highly polluted soil compared to free complex fungi (42.70%). Furthermore, CFI-RHB/SA maintained a stable removal efficiency (> 60%) in soils with higher concentrations of diesel pollution. This is attributed to the key role of fusarium and penicillium in the removal of diesel pollutants. Moreover, the integration of biochar amplifies microbial metabolic capacity, consequently bolstering dehydrogenase (DHA) activity within the soil matrix. Considering that DHA reflects the metabolic reaction of soil microorganisms to diesel oil, increased DHA activity indicates improved efficiency in the oxidative degradation of diesel oil, expediting the transfer of hydrogen atoms from organic substances to specific binding sites.
Li et al. investigated a biological carrier constructed from 10%-PVA, 0.5%-SA, and 5%-powdered activated carbon (PAC) for purifying soil contaminated by polycyclic aromatic hydrocarbons (PAHs)[48]. The results indicated that the carrier exhibited no significant cytotoxicity to the immobilized cells, and the live cell population of the immobilized bacteria was 1.86 × 1010 g-1. Furthermore, the biodegradation rates of phenanthrene and pyrene reached 87.0% and 75.4%, respectively, which is twice as high as the rates observed without the use of biological carriers.
Chen et al. utilized Enteromorpha prolifera biochar to immobilize a Bacillus cereus WHX-1 reducing strain (WBC), enhancing its Cr (VI) reduction activity, which was subsequently applied to the remediation of Cr-contaminated soil[49]. Experimental results indicated that incorporating WBC elevated the organic carbon content and cation exchange capacity of the soil, decreased the redox potential and bulk density, and markedly enhanced the physicochemical properties of Cr-contaminated soil. Remarkably, 94.22% of Cr (VI) was reduced to Cr (III), representing a 63.38% increase compared to the control group. To elucidate the removal mechanism, scanning electron microscope (SEM) and FTIR analyses revealed that the biochar surface was rough and porous, containing numerous functional groups, such as carboxyl, O-P-O, and amide I, which facilitate the adhesion of biochar to microorganisms. Furthermore, oxygen-containing functional groups act as electron donors, partially reducing Cr (VI). Additionally, biochar supplies nutrients to microorganisms, promoting their growth. Consequently, as depicted in Figure 3, the removal mechanism primarily involves biochar-enriched microbial colonies, thereby forming a stable microbial system in Cr-contaminated soil. This stable microbial aggregation system enhances the reduction of Cr (VI) and decreases the bioavailability of Cr, thereby achieving effective soil remediation.
Figure 3. Remediation mechanism of biochar-based Cr-reducing bacteria on Cr-contaminated soil plants.
The remediation of soil co-contaminated by organic pollutants and heavy metals is a significant challenge. Li et al. demonstrate the potential of using corncob biochar to immobilize Citrobacter strains (IM) for the remediation of soil co-contaminated by petroleum hydrocarbon and heavy metal nickel (Ni)[50]. The results indicated that the degradation rate of petroleum hydrocarbons by solid IM was 45.52%, which was significantly higher than that of free bacteria (30.15%) and biochar alone (25.92%). Importantly, no carcinogenic nickel sulfide was detected after the remediation process in IM. Additionally, the level of soil dehydrogenase activity of IM (0.3956 μg·mL-1·h-1·g-1) was higher than that of free bacteria
Application of microbial-carbon-based materials for removal of pollutant
Carrier material | Microbes | Contaminants | Initial concentrate | Reaction conditions | Removal capacity | Medium | Scale | Ref. |
Rice hull | Bacillus H27 | Chlorpyrifos | 25 mg/L | Time =7 days T = 25 °C Dose = 0.1 g | 97.40% | Water | Lab | [51] |
Rice husk | Aeromonas veronii | Chlorpyrifos | 30 mg/L | Time = 24 h T = 30 °C Dose = 1.375 g/L | 96.25% | Water | Lab | [52] |
10 mg/kg | Time = 42 days T = 30 °C Dose = 2 g/kg | 92.4% | Soil | Lab | ||||
Wheat straw | Serratia, Ralstonia, and Enterobacter | Phenol | 1,300 mg/L | Time = 72 h T = 30 °C Dose = 0.6% (w/v) | 82.4% | Water | Lab | [53] |
PFBCM | Pseudomonas aeruginosa | Phenanthrene | 80 mg/L | Time = 12 h T = 30 °C pH = 7 | 50% | Water | Lab | [54] |
41 mg/kg | Time = 6 days T = 30 °C Dose = 2 g | 30% | Soil | Lab | ||||
Enteromorpha prolifera | Bacillus cereus | Cr (VI) | 50 mg/kg | pH = 7.1 | 94.22% | Soil | Lab | [49] |
Rice straw | Bacillus cereus RC-1 | Cd2+ | 120 mg/L | pH = 6.0 Time = 20 h | 93.02 mg/g | Water | Lab | [55] |
Sewage sludge | 68.02 mg/g | |||||||
Chicken manure | 63.95 mg/g | |||||||
Maize straw | Sphingomonas sp. DZ3 | 4-bromodiphengl ether | 800 mg/L | pH = 7.0 Time = 24 h Dose = 0.1 g T = 40 °C | 50.23 mg/g | Water | Lab | [56] |
PVA/SA/biochar | Serratia marcescens | P-cresol | 500 mg/L | pH = 7.0 Time = 3 h T = 30 °C | 86.1% | Water | Lab | [57] |
Chitosan-biochar | Compound bacteria | Oil | 7,881 mg/kg | pH = 7.0 Time = 60 days T = 35 °C Dose = 2.0 g Salinity 1 MS | 45.82% | Soil | Lab | [58] |
Bamboo charcoal | Degrading bacteria | Nonylphenol | 50 mg/L | Time = 24 days | 93.95% | Water | Lab | [59] |
Activated carbon | Olivibacter jilunii | Total nitrogen | 249.7 mg/L | Time = 6 days | 97.4% | Water | Lab | [60] |
Total phosphorus | 19.7 mg/L | Time = 6 days | 96.1% | Water | Lab | |||
COD | 258 mg/L | Time = 6 days | 98.0% | Water | Lab | |||
Ammonia | 221 mg/L | 100% | ||||||
Granular activated carbon | Mycobacterium sp. | TMAH | 1.998 mg/L | 100% | Water | Lab | [61] | |
DOC | 4.392 mg/L | 78.8% | Water | Lab | ||||
SA/GO | Bacillus C5 | Crude oil | 8,000 mg/kg | Time = 30 days pH = 6.7 Dose = 1.0 kg | 64.92% | Soil | Lab | [62] |
GO | Paracoccus aminovorans | PAHs | Time = 35 days Dose = 0.9 g T = 30 °C | 62.86% | Soil | Lab | [63] |
In summary, these studies underscore the significant potential of immobilized microorganisms supported by carbon-based materials in the removal of various pollutants from soil and wastewater. It is a promising approach toward more sustainable and efficient environmental remediation strategies.
CONCLUSION AND FUTURE PERSPECTIVES
The application of immobilized microorganisms on carbon-based substrates has demonstrated considerable efficacy in the remediation of contaminated soil and water. This strategy harnesses the innate pollutant-degrading capabilities of microorganisms and the adsorption properties of carbon-based materials, yielding a more effective and potentially sustainable solution for environmental remediation. Immobilization techniques can augment the growth stability of microbial cells and extend their tolerance to endure harsh environments. Nevertheless, immobilization techniques encounter several challenges, such as operational complexity, biochar stability, environmental heterogeneity, limitations in mass transfer from the material surface to the immobilized microorganism, and the risk of thick biofilm formation that may hinder efficacy. Additionally, many microorganisms exhibit low tolerance to high concentrations of pollutants, which can result in suboptimal remediation outcomes and prolonged remediation cycles. Furthermore, environmental factors can also influence the effectiveness of immobilized microorganisms. Hence, the selection of novel, effective, and low-cost carbon carriers remains a significant challenge. Biochar and activated carbon are extensively utilized for cell immobilization due to their low toxicity and abundant availability. However, the underlying mechanisms of biochar and activated carbon immobilized bacteria composites in wastewater treatment and soil remediation require further exploration.
Furthermore, microbial immobilization utilizing carbon-based materials represents an advanced technological approach with distinct phases of implementation, each characterized by specific objectives and methodologies. At the laboratory scale, research focuses on the development and optimization of the immobilization technique. This involves conducting experiments with small quantities of carbon-based materials and microorganisms to evaluate key parameters such as microbial activity, substrate interaction, and process efficiency. The outcomes of these studies provide essential data for refining the technology and formulating hypotheses for subsequent stages. Field tests then transition the technology from controlled environments to practical settings, where pilot-scale systems or small-scale trials are employed to simulate environmental conditions. These tests are critical for assessing the stability and performance of the immobilized microorganisms under varied environmental factors, as well as addressing practical challenges related to material durability and microbial viability. The final stage of implementation involves the actual use of the technology in commercial or large-scale applications. This phase requires scaling up the technology, integrating it into existing industrial or environmental systems, and implementing continuous monitoring to ensure operational effectiveness and economic feasibility. The successful transition from laboratory research to field testing and commercial deployment is contingent upon overcoming challenges such as material costs, and integration with existing infrastructure.
DECLARATIONS
Authors’ contributions
Conceptualization, investigation, methodology, writing - original draft, supervision, formal analysis: Wang L
Writing - review and editing, methodology, supervision, project administration, funding acquisition, resources: Cheng D
Review and editing, methodology, resources: Liu X
Writing - review and editing, methodology, conceptualization, supervision, resources: Ye Y
Availability of data and materials
Not applicable.
Financial support and sponsorship
This research was supported by The Taishan Scholars Program of Shandong Province (tsqn202211157); Shandong Excellent Youth Science Fund Project (Overseas) (2023HWYQ-077); Project of Shandong Province Higher Educational Young Innovative Talent Introduction and Cultivation Team [Wastewater Treatment and Resource Innovation Team] (ZXQT20211216001); and National Natural Science Foundation of China (No. 52300210).
Conflicts of interest
Cheng D is a Junior Editorial Board member of the journal Water Emerging Contaminants & Nanoplastics and the Guest Editor of the Special Issue titled “Elimination of Emerging Contaminants and Micro(nano)plastics in the Anthropogenic Water Cycle”, while the other authors have declared that they have no conflicts of interest.
Ethical approval and consent to participate
Not applicable.
Consent for publication
Not applicable.
Copyright
© The Author(s) 2024.
REFERENCES
1. Jiang G. Preface to the special topic on environmental pollution and health risk. Natl Sci Rev 2016;3:409.
2. Zamora-Ledezma C, Negrete-Bolagay D, Figueroa F, et al. Heavy metal water pollution: a fresh look about hazards, novel and conventional remediation methods. Environ Technol Innov 2021;22:101504.
3. Li Q, Wen D, Qin C, Qian Y, Fu R, Lin S. Physical, chemical, biological, and synergistic technologies for remediation of pesticide-contaminated soil. Rev Environ Contam T 2024;262:7.
4. Gannett M, DiTommaso A, Sparks JP, Kao-Kniffin J. Microbial nitrogen immobilization as a tool to manage weeds in agroecosystems. Agr Ecosyst Environ 2024;366:108904.
5. Cao Y, Wang L, Wang Y, et al. Functional fungal pellets self-immobilized by mycelium fragments of Irpex lacteus WRF-IL for efficient degradation of sulfamethazine as the sole carbon source. Bioresour Technol 2023;385:129376.
6. Zhang J, Li F, Liu D, Liu Q, Song H. Engineering extracellular electron transfer pathways of electroactive microorganisms by synthetic biology for energy and chemicals production. Chem Soc Rev 2024;53:1375-446.
7. Wu P, Wang Z, Bhatnagar A, et al. Microorganisms-carbonaceous materials immobilized complexes: synthesis, adaptability and environmental applications. J Hazard Mater 2021;416:125915.
8. Jiang Y, Yang F, Dai M, et al. Application of microbial immobilization technology for remediation of Cr(VI) contamination: a review. Chemosphere 2022;286:131721.
9. Zhang J, Yang X, Wang S, et al. Immobilization of zinc and cadmium by biochar-based sulfidated nanoscale zero-valent iron in a co-contaminated soil: performance, mechanism, and microbial response. Sci Total Environ 2023;902:165968.
10. Yuan J, Liu Q, Chen Z, et al. Organic amendments perform better than inorganic amendments in reducing the absorption and accumulation of cadmium in lettuce. Environ Sci Pollut Res Int 2023;30:117277-87.
11. Nor Ashikin NAL, Mohd Fuzi SFZ, Abdul Manaf SA, Abdul Manas NH, Md Shaarani@ Md Nawi S, Md Illias R. Optimization and characterization of immobilized E. coli for engineered thermostable xylanase excretion and cell viability. Arab J Chem 2022;15:103803.
12. Huang F, Dong F, Chen L, et al. Biochar-mediated remediation of uranium-contaminated soils: evidence, mechanisms, and perspectives. Biochar 2024;6:308.
13. Han J, Zhang J, Meng J, et al. Characterization of modified rice straw biochar in immobilizing Bacillus subtilis 168 and evaluation on its role as a novel agent for zearalenone-removal delivery. J Hazard Mater 2023;453:131424.
14. Zhang S, Wang J. Removal of chlortetracycline from water by immobilized Bacillus subtilis on honeysuckle residue–derived biochar. Water Air Soil Pollut 2021;232:236.
15. Zhang S, Wang J. Removal of chlortetracycline from water by Bacillus cereus immobilized on Chinese medicine residues biochar. Environ Technol Innov 2021;24:101930.
16. Zheng Z, Ali A, Su J, Zhang S, Su L, Qi Z. Biochar fungal pellet based biological immobilization reactor efficiently removed nitrate and cadmium. Chemosphere 2022;296:134011.
17. Xiang X, Yi X, Zheng W, et al. Enhanced biodegradation of thiamethoxam with a novel polyvinyl alcohol (PVA)/sodium alginate (SA)/biochar immobilized Chryseobacterium sp H5. J Hazard Mater 2023;443:130247.
18. Zeng Z, Xiao J, Li M, Wu J, Zhang T. Degradation of phenol by immobilized Alcaligenes faecalis strain JH1 in Fe3O4-modified biochar from pharmaceutical residues. Water 2023;15:4084.
19. Zhu P, Zhuo S, Zhang W, Ying X, Huang J, Li X. Performance and application of microbial fuel cells with sodium alginate/agar/activated carbon composite as efficient biocompatible anode. J Appl Polym Sci 2023;140:e53999.
20. Lin JE, Wang HY, Hickey RF. Use of coimmobilized biological systems to degrade toxic organic compounds. Biotechnol Bioeng 1991;38:273-9.
21. Annadurai G, Juang RS, Lee DJ. Biodegradation and adsorption of phenol using activated carbon immobilized with Pseudomonas putida. J Environ Sci Heal A 2002;37:1133-46.
22. Maharaja P, Mahesh M, Patchaimurugan N, Swarnalatha S, Sekaran G. Treatment of slaughterhouse wastewater by integrated anaerobic/aerobic bioreactors loaded with immobilized nanoporous activated carbon. J Am Leather Chem As 2021;116:303-11. Available from: https://www.researchgate.net/publication/354311129_Treatment_of_Slaughterhouse_Wastewater_by_Integrated_AnaerobicAerobic_Bioreactors_Loaded_with_Immobilized_Nanoporous_Activated_Carbon. [Last accessed on 18 Aug 2024]
23. Yang J, Xu SY, Zhang T, et al. A dual bacterial alliance removed erythromycin residues by immobilizing on activated carbon. Bioresour Technol 2023;384:129288.
24. Zhai X, Liu X, Dong H, Lin M, Zheng X, Yang Q. Implementation of cytochrome c proteins and carbon nanotubes hybrids in bioelectrodes towards bioelectrochemical systems applications. Bioprocess Biosyst Eng 2024;47:159-68.
25. Kim K, Moon D, Jo M, Ahn H. Carbon nanotube-interlocked Si/CNF self-supporting electrode using continuable spraying architecture system for flexible lithium-ion batteries. Appl Surf Sci 2024;656:159663.
26. Alagöz D, Toprak A, Yildirim D, Tükel SS, Fernandez-Lafuente R. Modified silicates and carbon nanotubes for immobilization of lipase from Rhizomucor miehei: effect of support and immobilization technique on the catalytic performance of the immobilized biocatalysts. Enzyme Microb Technol 2021;144:109739.
27. Akyilmaz E, Canbay E, Dinçkaya E, Güvenç C, Yaşa İ, Bayram E. Simultaneous determination of epinephrine and dopamine by using Candida tropicalis yeast cells immobilized in a carbon paste electrode modified with single wall carbon nanotube. Electroanalysis 2017;29:1976-84.
28. Boshagh F, Rostami K, Moazami N. Biohydrogen production by immobilized Enterobacter aerogenes on functionalized multi-walled carbon nanotube. Int J Hydrogen Energ 2019;44:14395-405.
29. Perchikov RN, Provotorova DV, Kharkova AS, et al. Bioanalytical system for determining the phenol index based on Pseudomonas putida BS394(pBS216) bacteria immobilized in a redox-active biocompatible composite polymer “bovine serum albumin-ferrocene-carbon nanotubes”. Polymers 2022;14:5366.
30. Manzoor Q, Farrukh MA, Sajid A. Optimization of lead (II) and chromium (VI) adsorption using graphene oxide/ZnO/chitosan nanocomposite by response surface methodology. Appl Surf Sci 2024;655:159544.
31. Zhao C, Liu J, Deng Y, et al. Uranium(VI) adsorption from aqueous solutions by microorganism-graphene oxide composites via an immobilization approach. J Clean Prod 2019;236:117624.
32. Liu Y, Li Y, Yuan X, Ren R, Lv Y. A self-prepared graphene oxide/sodium alginate aerogel as biological carrier to improve the performance of a heterotrophic nitrifier. Biochem Eng J 2021;171:108027.
33. Yong Y, Liao Z, Sun J, Zheng T, Jiang R, Song H. Enhancement of coulombic efficiency and salt tolerance in microbial fuel cells by graphite/alginate granules immobilization of Shewanella oneidensis MR-1. Process Biochem 2013;48:1947-51.
34. Li Y, Sun J, Wang J, et al. A microbial electrode based on the co-electrodeposition of carboxyl graphene and Au nanoparticles for BOD rapid detection. Biochem Eng J 2017;123:86-94.
35. Zhang S, Wang J, Wang S, Leng S. Effective removal of chlortetracycline and treatment of simulated sewage by Bacillus cereus LZ01 immobilized on erding medicine residues biochar. Biomass Conv Bioref 2024;14:2281-91.
36. Yu T, Wang L, Ma F, Wang Y, Bai S. A bio-functions integration microcosm: self-immobilized biochar-pellets combined with two strains of bacteria to remove atrazine in water and mechanisms. J Hazard Mater 2020;384:121326.
37. Manikandan SK, Nair V. Pseudomonas stutzeri immobilized sawdust biochar for nickel ion removal. Catalysts 2022;12:1495.
38. Mittal H, Alfantazi A, Alhassan SM. Recent developments in the adsorption of uranium ions from wastewater/seawater using carbon-based adsorbents. J Environ Chem Eng 2024;12:111705.
39. Manoj D, Saravanan R, Raji A, Thangamani A. Carbon-based microelectrodes for environmental remediation: progress, challenges and opportunities. Carbon Lett 2023;33:1485-93.
40. González-González RB, Martínez-Zamudio LY, Hernández JAR, González-Meza GM, Parra-Saldívar R, Iqbal HMN. Pharmaceutical pollution fingerprinting and waterbodies remediation using waste-derived carbon dots as sustainable advanced nanomaterials. Environ Res 2023;238:117180.
41. Dhiman P, Goyal D, Rana G, et al. Recent advances on carbon-based nanomaterials supported single-atom photo-catalysts for waste water remediation. J Nanostruct Chem 2024;14:21-52.
42. An Q, Jin N, Deng S, et al. Ni(II), Cr(VI), Cu(II) and nitrate removal by the co-system of Pseudomonas hibiscicola strain L1 immobilized on peanut shell biochar. Sci Total Environ 2022;814:152635.
43. Huang F, Li K, Wu R, Yan Y, Xiao R. Insight into the Cd2+ biosorption by viable Bacillus cereus RC-1 immobilized on different biochars: roles of bacterial cell and biochar matrix. J Clean Prod 2020;272:122743.
44. Ouyang X, Yin H, Yu X, et al. Enhanced bioremediation of 2,3’,4,4’,5-pentachlorodiphenyl by consortium GYB1 immobilized on sodium alginate-biochar. Sci Total Environ 2021;788:147774.
45. Lu L, Li A, Ji X, Yang C, He S. Removal of acenaphthene from water by Triton X-100-facilitated biochar-immobilized Pseudomonas aeruginosa. RSC Adv 2018;8:23426-32.
46. Guo S, Yang H, Sun Q, et al. Evaluation of a novel carbon-based micro-nano zero-valent iron composite for immobilization of heavy metals in soil. J Environ Chem Eng 2023;11:109740.
47. Yin C, Yan H, Cao Y, Gao H. Enhanced bioremediation performance of diesel-contaminated soil by immobilized composite fungi on rice husk biochar. Environ Res 2023;226:115663.
48. Li P, Wang X, Stagnitti F, et al. Degradation of phenanthrene and pyrene in soil slurry reactors with immobilized bacteria Zoogloea sp. Environ Eng Sci 2005;22:390-9.
49. Chen Y, Wu H, Sun P, et al. Remediation of chromium-contaminated soil based on Bacillus cereus WHX-1 immobilized on biochar: Cr(VI) transformation and functional microbial enrichment. Front Microbiol 2021;12:641913.
50. Li X, Wang Y, Luo T, Ma Y, Wang B, Huang Q. Remediation potential of immobilized bacterial strain with biochar as carrier in petroleum hydrocarbon and Ni co-contaminated soil. Environ Technol 2022;43:1068-81.
51. Liu C, Wen S, Li S, et al. Enhanced remediation of chlorpyrifos-contaminated soil by immobilized strain Bacillus H27. J Environ Sci 2024;144:172-84.
52. Manikandan S, Mariyam A, Gowda NK, Singh A, Nair V. Mechanistic understanding of biochar-bacteria system for enhanced chlorpyrifos bioremediation in water and soil medium. Chem Eng J 2024;483:149119.
53. Dong J, Xu L, Liu Y, Ren L, Yuan K. Biochar enhances microbial degradation of phenol in water: response surface optimization. Biochem Eng J 2024;201:109145.
54. Wang K, Sun Y, Chen D, et al. Enhanced remediation of phenanthrene in water and soil by novel biochar-immobilized bacterial microspheres. Chem Eng J 2023;462:141932.
55. Deng M, Li K, Yan YJ, Huang F, Peng D. Enhanced cadmium removal by growing Bacillus cereus RC-1 immobilized on different magnetic biochars through simultaneous adsorption and bioaccumulation. Environ Sci Pollut Res Int 2022;29:18495-507.
56. Du J, Sun P, Feng Z, Zhang X, Zhao Y. The biosorption capacity of biochar for 4-bromodiphengl ether: study of its kinetics, mechanism, and use as a carrier for immobilized bacteria. Environ Sci Pollut Res Int 2016;23:3770-80.
57. Jaiswal VK, Sonwani RK, Singh RS. Assessment of enhanced p-cresol biodegradation by encapsulating pre-immobilised Serratia marcescens strain HL 1 on tea waste biochar into polyvinyl alcohol/sodium alginate matrix. Biochem Eng J 2023;199:109046.
58. Liu Q, Wang Y, Sun S, et al. A novel chitosan-biochar immobilized microorganism strategy to enhance bioremediation of crude oil in soil. Chemosphere 2023;313:137367.
59. Lou L, Huang Q, Lou Y, Lu J, Hu B, Lin Q. Adsorption and degradation in the removal of nonylphenol from water by cells immobilized on biochar. Chemosphere 2019;228:676-84.
60. Huang J, Xiao Y, Chen B. Nutrients removal by Olivibacter jilunii immobilized on activated carbon for aquaculture wastewater treatment: ppk1 gene and bacterial community structure. Bioresour Technol 2023;370:128494.
61. Yang B, Oh H, Lee Y, Jung J, Jahng D. Low-strength electronic wastewater treatment using immobilized cells of TMAH-degrading bacterium followed by activated carbon adsorption. Desalin Water Treat 2015;54:3639-45.
62. Liu Q, Chen H, Su Y, et al. Enhanced crude oil degradation by remodeling of crude oil-contaminated soil microbial community structure using sodium alginate/graphene oxide/Bacillus C5 immobilized pellets. Environ Res 2023;223:115465.
Cite This Article

How to Cite
Wang, L.; Cheng D.; Liu X.; Ye Y. Utilizing microorganisms immobilized on carbon-based materials for environmental remediation: a mini review. Water. Emerg. Contam. Nanoplastics. 2024, 3, 19. http://dx.doi.org/10.20517/wecn.2024.14
Download Citation
Export Citation File:
Type of Import
Tips on Downloading Citation
Citation Manager File Format
Type of Import
Direct Import: When the Direct Import option is selected (the default state), a dialogue box will give you the option to Save or Open the downloaded citation data. Choosing Open will either launch your citation manager or give you a choice of applications with which to use the metadata. The Save option saves the file locally for later use.
Indirect Import: When the Indirect Import option is selected, the metadata is displayed and may be copied and pasted as needed.
About This Article
Special Issue
Copyright
Data & Comments
Data
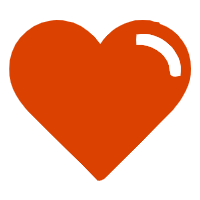
Comments
Comments must be written in English. Spam, offensive content, impersonation, and private information will not be permitted. If any comment is reported and identified as inappropriate content by OAE staff, the comment will be removed without notice. If you have any queries or need any help, please contact us at support@oaepublish.com.