Effect of exposure to traffic-related air pollution on liver function during the compensation period for cirrhosis: a 4-year follow-up cohort study
Abstract
The increasing number of motor vehicles due to economic and urbanization development has raised concerns about the impact of traffic-related air pollution on human health. Research on the impact of traffic-related pollution on populations, particularly in individuals with liver cirrhosis, is currently lacking. In this study, we utilized data from compensated cirrhosis patients at the Second People’s Hospital in Tianjin, China, to investigate the effects of traffic-related air pollution exposure on liver function-related blood indicators. A multiple linear regression model was applied to analyze the exposure to six air pollutants as well as the proximity of patients’ residential (or work) addresses to traffic arteries (main roads, secondary roads, highways). The results indicated a significant correlation between traffic-related air pollution and certain levels of liver function. Specifically, PM2.5 exposure was positively correlated with prothrombin time [PT, P < 0.05, 95%CI = (0.119, 0.424)] and international normalized ratio [INR,
Keywords
INTRODUCTION
As people become increasingly concerned about health issues, air pollution as a significant factor in causing diseases has garnered growing public attention[1]. According to the report from the World Health Organization (WHO) Global Air Quality Guidelines (AQGs), as of 2019, 99% of the global population resides in areas that fail to meet the AQGs set by the WHO[2]. According to the China Ecological Environment Status Bulletin for 2022, released by the Ministry of Ecology and Environment, only 62.8% of urban environmental air quality in 339 prefecture-level and above cities across the nation meets the standards. Air pollution exerts a significant adverse impact on public health. It is estimated that in 2019, environmental air pollution led to 4.2 million premature deaths globally, emerging as the fourth leading cause of premature mortality[3]. Data from the Global Burden of Disease (GBD) show that in 2021, air pollution is a major secondary risk factor for causing disability-adjusted life years (DALYs) among all environmental and occupational risks, resulting in 236 million DALYs and 8.08 million death cases[4].
Traffic-related air pollution is one of the main sources of air pollution globally[5]. It encompasses a complex mixture of various gases and particulate matter (PM), including nitrogen oxides (NOx), elemental carbon (EC), carbon monoxide (CO), hydrocarbons (HC), and PM. These pollutants are typically emitted directly from vehicle exhausts but can also originate from non-exhaust sources such as fuel evaporation, dust resuspension, brake and tire wear, and road surface abrasion[6,7]. As rapid socio-economic development and urbanization continue, urban transportation networks are expanding, leading to the growing demand for motor vehicles. Statistics show that between 2009 and 2018, the number of motor vehicles nationwide rose from 170 to 327 million, with an average annual growth rate of 7.5%. Among these motor vehicles, cars are the primary source of air pollution, accounting for over 90% of total emissions of CO, HC, NOx, and PM. Despite efforts on regulations as well as technological advancements in motor vehicles, emissions from traffic sources exhibit an overall downward trend but remain at relatively high levels.
Exposure to air pollution has a significant detrimental impact on health, particularly regarding blood biomarkers. Epidemiological studies indicate a strong association between air pollution and the risk of developing cardiovascular diseases[8,9], respiratory conditions[10], neurological disorders[11,12], and metabolic dysfunctions[13]. Notably, research has shown that air pollution exposure can significantly affect liver function-related blood markers. For instance, Markevych et al. established a significant correlation between gamma-glutamyl transferase (GGT) levels and PM2.5 exposure, with GGT serving as a marker of oxidative stress[14]. Qiu et al. discovered positive associations between exposure levels of PM2.5, PM10, nitrogen dioxide (NO2), sulfur dioxide (SO2), CO, and ozone (O3) and alanine aminotransferase (ALT). Specifically, PM10 exposure was positively correlated with aspartate aminotransferase (AST), while CO and O3 exposure exhibited a negative correlation with AST[15]. Furthermore, Kim et al. identified a significant relationship between exposure to air pollutants and the logarithmic increase in ALT and AST levels, particularly noting that long-term exposure to PM10 and CO is a risk factor for elevated liver function in alcoholic adults[16]. Their analysis of panel data from Korean individuals aged 60 and above revealed a close association between short-term exposure to air pollutants (including PM2.5, NO2, and O3) and elevated liver enzyme levels[17]. Additionally, Tan et al. observed a significant increase in albumin, blood urea nitrogen (BUN), and creatinine levels among traffic police personnel with long-term exposure to traffic pollution[18]. These studies indicate that prolonged exposure to air pollutants leads to a significant increase in blood markers associated with liver function, which indirectly highlights the potential risk of air pollution causing abnormal liver function and even liver diseases.
Numerous toxicological studies have also confirmed that air pollution has significant adverse effects on health. Inhaled PM can penetrate deep into the lungs and enter the circulatory system, where it disperses into distant organs such as the heart, spleen, and liver[19], potentially triggering systemic oxidative stress and inflammatory responses[20]. Studies have identified a significant correlation between air pollution and the incidence of metabolic syndrome[21-23], with the liver, a crucial metabolic organ, being directly affected by PM2.5 exposure. This exposure can lead to abnormalities in lipid metabolism, inflammatory responses, and insulin resistance[24,25], ultimately increasing the risk of metabolic-related diseases such as fatty liver, liver cirrhosis, and even liver cancer[26,27]. Therefore, air pollution exacerbates the disease burden on the metabolic system, particularly the liver, posing an increasingly severe threat to public health.
At present, efforts are being made to reveal the complex mechanisms of air pollution-induced liver injury. Mouse experiments have shown that prolonged exposure to PM2.5 for over 10 weeks can lead to fatty liver, inflammation, and fibrosis, and impair the liver’s glucose metabolism[28]. Inhaling PM2.5 can also trigger endoplasmic reticulum stress in lung and liver tissues, activate the unfolded protein response, and subsequently affect the function of the RAW264.7 macrophage cell line[29]. Moreover, Dybdahl et al. found that mice exposed to diesel engine exhaust particles experience oxidative stress, DNA damage, and apoptosis of liver cells[30]. Summarizing the known mechanisms of liver damage caused by air pollution, it can be broadly described as: air pollutants induce liver cell damage, liver oxidative stress, and inflammatory reactions, which, over time, can ultimately lead to liver pathology.
Current research highlights the potential adverse effects of air pollution on liver function and underscores the necessity for further studies and public health interventions to mitigate these risks. However, much of the existing literature focuses primarily on general air pollution, lacking in-depth exploration of the impacts associated with specific sources, such as traffic-related emissions. Additionally, epidemiological studies have predominantly targeted relatively healthy populations, leaving a significant gap in understanding how air pollution affects individuals with pre-existing liver conditions. Addressing this research gap is essential for a comprehensive understanding of the effects of air pollution across various health profiles.
In this study, we utilized data from a subset of compensated cirrhosis patients at the Second People’s Hospital in Tianjin, and employed a multiple linear regression model to assess the impact of exposure to various air pollutants, including PM (PM10, PM2.5), SO2, NO2, CO, and O3, as well as the proximity of patients’ residential or work addresses to traffic arteries (main roads, secondary roads, highways), on liver function-related blood indicators. This research can provide a basis for a comprehensive evaluation of the health risks posed by traffic-related air pollution.
EXPERIMENTAL
Study population and study design
This study was a retrospective cohort study. The subjects of this study were patients with decompensated cirrhosis who were treated in Tianjin Second People’s Hospital from November 2, 2015, to December 31, 2016, and received a three-year follow-up survey before December 31, 2019.
The specific inclusion criteria were as follows: (1) Age of 18-75 years old; (2) Patients with cirrhosis diagnosed before the cohort study according to histological and/or clinical diagnosis (clinical diagnosis of irregular liver morphology, nodular appearance, and abnormal liver function on ultrasound, CT, or MRI). Other imaging findings include hepatomegaly, splenomegaly, and portal hypertension. Patients with post-hepatitis B cirrhosis receiving standardized antiviral therapy; Patients with post-hepatitis C cirrhosis who achieved sustained virologic response to DAA antiviral therapy; (3) Patients without primary or metastatic liver cancer and no history of decompensated cirrhosis (variceal bleeding, ascites, hepatic encephalopathy); (4) Complete 3-year follow-up; (5) Relevant indicators tested during follow-up, including complete blood routine, coagulation function, liver function, blood glucose, abdominal ultrasound, CT or MRI, LSM, electronic endoscopy, and endoscopic ultrasonography; (6) No relevant treatment affecting blood indicators during follow-up, such as prophylactic treatment for esophageal and gastric varices, including: Endoscopic treatment (endoscopic ligation or endoscopic sclerotherapy), oral non-selective β-blockers, interventional radiation therapy (TIPS, balloon retrograde transvenous occlusion), or surgical treatment (splenectomy and shunt surgery). This study has been approved by the Ethics Committee of Second People’s Hospital of Tianjin ([2015]15).
After screening, a total of 38 patients were eligible. Baseline clinical data were collected, including age, sex, weight, height, alcohol consumption, smoking status, and personal and family history.
We organized a PICOT table related to this study to display the specific design of the research, as shown in Table 1.
The PICOT table of this study
P (population) | Patients with decompensated cirrhosis who were treated at Tianjin Second People’s Hospital from November 2, 2015, to December 31, 2016, and received a three-year follow-up survey before December 31, 2019 |
I (intervention/exposure) | The exposure of patients to traffic-related air pollution during the follow-up period |
C (comparison) | This study did not set up a control group. As it is a retrospective cohort study, the main objective is to describe and analyze the health outcomes of patients with decompensated cirrhosis during the follow-up period, with a focus on comparing changes between the patients’ baseline data and subsequent observational data |
O (outcome) | The outcome indicators of the study include several liver function-related blood parameters (such as ALT, AST, GGT, etc.) and other relevant blood parameters (such as CK, PLT, etc.). These indicators can reflect the liver health status of the patients and the changes during the follow-up period |
T (type of study) | This study is a retrospective cohort study that primarily analyzes and evaluates past case data. The time frame of the study design spans from the start date of treatment for the patients to the end of the follow-up period |
Blood collection and measurement of liver function and other blood parameters
Blood parameters for each patient were measured during their final follow-up visit. The blood parameters determined in this study included liver function-related indicators [AST (U/L), ALT (U/L), cholinesterase (CHE, U/L), GGT (U/L)], and other relevant blood parameters [creatine kinase (CK, U/L), BUN (mmol/L), cholesterol (CHO, mmol/L), triglyceride (TG, mmol/L), prothrombin time (PT, s), international normalized ratio (INR), serum ferritin (SF, μg/L), hemoglobin (Hb, g/L), and platelets (PLT, /L)]. Blood samples were collected in the morning to avoid variations due to time fluctuations throughout the day. After centrifugation of the blood samples (2,000 rpm, 15 min), all blood parameters were analyzed in the hospital clinical laboratory using a biochemical analyzer and commercial assay kits, strictly following the laboratory requirements and the instructions of the assay kit manufacturer.
Assessment of air pollution exposure and traffic exposure
The data on the concentration of 6 pollutants from 23 monitoring sites (including national and municipal monitoring sites) in Tianjin from 2015 to 2020 were obtained from the Tianjin Ecological Environment Bureau. With a relatively sufficient number of monitoring sites, balanced distribution, and comprehensive spatial coverage, this study utilized a proximity model to assess patients’ levels of exposure to air pollutants. The proximity model estimates the exposure levels of study subjects based on the distance between air pollution sources and the study objects, with the patients’ addresses corresponding to the nearest national monitoring points to calculate their annual average exposure levels to air pollutants. Missing values were filled and assigned using the average values of air pollutants from the two nearest days, with missing values accounting for 4.1% of the air pollution data.
The assessment of traffic exposure primarily involved using maps to calculate the distance between the patients’ residential (or work) addresses and the main roads, secondary roads, and highways. In this study, the assessment of air pollution exposure and the intensity of traffic exposure was illustrated in Figure 1, where the gray area represented Tianjin, the blue dots represented patient addresses, the red triangles marked the locations of national monitoring points, and the dark lines represented traffic arteries.
Statistical analysis
In this study, the Chi-square test was used to assess the strength of the correlations among variables in the patient survey information, which is useful for subsequent regression model establishment and variable selection. Since some individual variables had small expected values, the Chi-square test was deemed inaccurate, and hence, the Fisher exact test was used instead to understand the relationships between the variables. The Chi-square test is commonly used to analyze the relationships between unordered categorical variables and can also be applied to the analysis of binary variables. However, this test can only analyze statistical significance and cannot reflect the strength of the relationship. The Fisher exact test, on the other hand, is often used to analyze the relationship between two binary variables and is more suitable for analyzing data with small sample sizes. Like the Chi-square test, it can only analyze statistical significance and cannot reflect the strength of the relationship.
We employed a multiple linear regression model to analyze the correlation between liver function, other blood indicators, and levels of traffic-related air pollution exposure. The stepwise regression method was used to select the model with the smallest Akaike information criterion (AIC) value. In cases where independent variables were highly correlated in the stepwise regression analysis model, they were sequentially removed until a final model was selected with the lowest AIC value and no correlation between variables. Additionally, the model was adjusted for potential confounding factors such as gender, body mass index (BMI), alcohol consumption, smoking, hypertension, coronary heart disease, diabetes, and fatty liver. The model establishment and analysis were conducted using R version 3.6.1, with a two-tailed P-value < 0.05 considered statistically significant.
Since the dependent variables following a normal distribution are more conducive to model establishment and analysis, the Shapiro-Wilk test was performed to determine if the liver function and other blood indicators, including CHE, CHO, Hb, and PLT, followed a normal distribution. For indicators that did not follow a normal distribution, a logarithmic transformation was applied, followed by a normality test. If normal distribution was still not achieved, the original data were used for analysis. In the analysis of liver function indicators, logarithmic transformations were applied to ALT, CK, BUN, and TG for analysis.
RESULTS
Air pollution and traffic exposure situation
The measured air pollution exposure levels in the study refer to the annual average concentrations of air pollutants for patients in the year prior to undergoing liver function tests. Table 2 displayed the annual average pollutant concentration data for patients’ liver function exposure. The annual average concentration of pollutants was calculated as the average of daily concentrations throughout the year, while the daily concentration was the arithmetic average of data from all monitoring sites on a given day. The data collection integrity and consistency at the monitoring sites comply with national environmental air quality monitoring standards, with O3 concentrations calculated as the daily maximum 8-hour average and other pollutants calculated as the 24-hour averages. During the study period, the annual average pollution levels of PM2.5 and PM10 to which patients were exposed were significantly higher than the secondary concentration limits stipulated in the Environmental Air Quality Standards (GB 3095-2012), whereas the annual average pollution levels of NO2 exceeded the secondary standards. The annual average concentration of SO2 did not exceed the secondary standard. Furthermore, since there are no specified annual average standards for CO and O3, CO used the secondary standard limit for the 24-hour average concentration as a reference, while O3 referred to the secondary standard limit for the daily maximum 8-hour average concentration. The comparison revealed that both CO and O3 did not exceed the secondary standard limits.
Descriptive statistics of patient’s annual average exposure to air pollutants
Air pollutant | Minimum | Median | Maximum | Mean | Ambient air quality standards (secondary standards) |
PM2.5 (μg/m3) | 57.542 | 75.563 | 88.29 | 74.088 | 35 |
PM10 (μg/m3) | 104.53 | 114.53 | 122.834 | 114.497 | 70 |
SO2 (μg/m3) | 15.748 | 20.244 | 25.645 | 20.488 | 60 |
NO2 (μg/m3) | 46.997 | 53.657 | 56.233 | 52.826 | 40 |
CO (mg/m3) | 1.242 | 1.52 | 1.634 | 1.486 | 4a |
O3 (μg/m3) | 45.885 | 58.038 | 67.808 | 58.001 | 160b |
In the statistical analysis of traffic exposure, the preference was to use patients’ home addresses for data collection; in cases where home addresses are unavailable, work addresses were used as a substitute. The traffic exposure intensity for 16 patients was calculated based on their work addresses. The assessment of traffic exposure intensity primarily relied on the distance between patients’ home addresses (or work addresses) and main roads, secondary roads, and highways. Table 3 provided a comprehensive description of the patients’ traffic exposure intensity levels.
Descriptive statistics of patients’ traffic exposure intensity
Traffic exposure | Minimum | Median | Maximum | Mean |
Distance from the secondary road (m) | 7 | 86 | 837 | 121.526 |
Distance from the main road (m) | 23 | 452 | 1,600 | 451.237 |
Distance from the highway (km) | 0.53 | 4.8 | 8.4 | 4.747 |
Descriptive analysis of patient characteristics
The statistical description of 39 cirrhotic patients who were followed up at Tianjin Second People’s Hospital from 2015 to 2020 was shown in Table 4. After excluding patients with missing home addresses (work addresses), survey information, follow-up period, and liver function data, 38 patients with good liver function were finally selected for the study. Among these patients, there were an equal number of male and female patients; the majority of patients had a BMI value between 18-30 (86.9%); most patients did not drink alcohol (68.4%), did not smoke (68.4%), and had no history of hypertension (84.2%), no history of coronary heart disease (94.7%), no history of diabetes (68.4%), and no fatty liver (84.2%).
Patient information collection
Characteristics | Value | |
Gender | Male | 19 (50.0%) |
Female | 19 (50.0%) | |
BMI | < 18 | 1 (2.6%) |
18-25 | 19 (50.0%) | |
15-30 | 14 (36.9%) | |
< 30 | 4 (10.5%) | |
Drinking | Yes | 12 (31.6%) |
No | 26 (68.4%) | |
Smoking | Yes | 12 (31.6%) |
No | 26 (68.4%) | |
Hypertension | Yes | 6 (15.8%) |
No | 32 (84.2%) | |
CHD | Yes | 2 (5.3%) |
No | 36 (94.7%) | |
Diabetes mellitus | Yes | 12 (31.6%) |
No | 26 (68.4%) | |
Fatty liver | Yes | 6 (15.8%) |
No | 32 (84.2%) |
Table 5 presented the basic characteristics of blood indicators related to liver cirrhosis patients in this study; some patients had missing data for certain indicators. After excluding extreme values of maximum and minimum in each group, it was observed from the mean values that the levels of GGT, PT, and SF in patients were significantly elevated, which was associated with the compensation period of liver cirrhosis. When cirrhosis occurs, liver function is impaired, resulting in elevated levels of GGT in the blood. Furthermore, liver dysfunction caused by liver cirrhosis may affect the synthesis and metabolism of ferritin and coagulation factors, potentially leading to increased SF levels and prolonged coagulation time.
Liver function-related blood indexes of patients
Blood index | Sample size | Minimum | Median | Maximum | Mean |
ALT (U/L) | 38 | 9 | 23 | 107 | 27.816 |
AST (U/L) | 38 | 12 | 21.5 | 92 | 28.316 |
CHE (U/L) | 23 | 2,343 | 7,142 | 11,543 | 7,171 |
GGT (U/L) | 38 | 13 | 30 | 375 | 52.579 |
CK (U/L) | 27 | 32 | 68 | 193 | 74.963 |
BUN (mmol/L) | 30 | 2.6 | 4.65 | 11.7 | 5.13 |
CHO (mmol/L) | 26 | 2.49 | 4.355 | 7.1 | 4.387 |
TG (mmol/L) | 26 | 0.56 | 1.05 | 2.16 | 1.216 |
PT (s) | 20 | 12.4 | 13.4 | 20.8 | 14.025 |
INR | 20 | 0.92 | 1.035 | 1.78 | 1.089 |
SF (μg/L) | 17 | 47 | 83 | 1,225 | 227.588 |
Hb (g/L) | 27 | 108 | 142 | 175 | 141.926 |
PLT (× 109/L) | 27 | 33 | 114 | 306 | 122.037 |
The results of the χ2 test or Fisher’s exact test [Table 6] indicated a strong correlation among the three independent variables of gender, alcohol consumption, and smoking. This was attributed to the higher proportion of males among patients with a history of smoking and alcohol consumption, with the majority having both a history of smoking and alcohol consumption.
Results of the correlation test for patient information variables
Characteristics | Gender | BMI | Drinking | Smoking | HTN | CHD | DM | Fatty liver |
Gender | 1.000 | 0.320 | < 0.001* | 0.002* | > 0.999 | > 0.999 | 0.295 | > 0.999 |
BMI | 1.000 | 0.459 | 0.682 | 0.327 | 0.061 | 0.138 | 0.232 | |
Drinking | 1.000 | < 0.001* | 0.357 | > 0.999 | 0.139 | 0.643 | ||
Smoking | 1.000 | 0.357 | > 0.999 | 0.139 | 0.643 | |||
HTN | 1.000 | > 0.999 | 0.643 | > 0.999 | ||||
CHD | 1.000 | > 0.999 | > 0.999 | |||||
DM | 1.000 | 0.357 | ||||||
Fatty liver | 1.000 |
Analysis of the correlation between exposure to traffic-related air pollution and liver function indicators
In the adjusted multiple linear regression model, the changes in certain liver function indicators of patients with liver cirrhosis were associated with exposure to traffic-related air pollution [Table 7]. In the model for CHE, the levels of CHE in patients were related to air pollutants NO2 and CO. The exposure concentration of NO2 was negatively correlated with CHE [P < 0.05, 95%CI = (-1,295.811, -45.806)], with a decrease of 670.809 in CHE for every 1 µg/m3 increase in NO2 concentration, while the exposure concentration of CO was positively correlated with CHE [P < 0.05, 95%CI = (15,276.644, 55,907.446)], with an increase of 35,592.045 in CHE levels for every 1 mg/m3 increase in CO concentration. In the models for ALT, AST, and GGT, there was no statistically significant correlation between the changes in liver function indicators and the concentration of traffic-related pollutants.
Regression analysis results of traffic-related air pollution and liver function-related blood indicators
β (95%CI) | ||||
ALT | AST | CHE | GGT | |
PM2.5 (μg/m3) | -2.21 (-4.64, 0.22) | -1.51 (-3.70, 0.68) | -317.53 (-740.01, 104.96) | -5.80 (-14.87, 3.27) |
PM10 (μg/m3) | 0.62 (-1.18, 2.42) | 0.55 (-1.14, 2.24) | -89.74 (-477.48, 298.00) | 1.77 (-4.75, 8.28) |
SO2 (μg/m3) | 3.50 (-1.87, 8.86) | 0.21 (-4.40, 4.83) | -585.19 (-1,614.97, 444.60) | 3.60 (-15.18, 22.39) |
NO2 (μg/m3) | 0.36 (-3.24, 3.95) | 1.65 (-1.70, 5.00) | -670.81 (-1,295.81, -45.81)* | 1.67 (-11.54, 14.87) |
CO (mg/m3) | -34.93 (-162.62, 92.76) | 2.63 (-132.68, 137.94) | 35,592.05 (15,276.64, 55,907.45)* | -40.42 (-590.16, 509.33) |
O3 (μg/m3) | 1.25 (-0.42, 2.91) | -0.49 (-1.92, 0.94) | -236.75 (-491.86, 18.36) | 0.16 (-5.40, 5.72) |
Distance from the secondary road (m) | 0.00 (-0.04, 0.04) | -0.00 (-0.04, 0.03) | 8.37 (3.78, 12.96)* | -0.01 (-0.13, 0.12) |
Distance from the main road (m) | 0.01 (-0.01, 0.04) | -0.02 (-0.04, -0.01)* | -1.89 (-5.17, 1.39) | -0.08 (-0.15, -0.01)* |
Distance from the highway (km) | -0.12 (-2.73, 2.50) | 0.76 (-1.58, 3.09) | 52.26 (-314.234, 418.744) | -0.41 (-9.01, 8.20) |
Table 7 showed that certain liver function indicators of patients with liver cirrhosis were associated with exposure to traffic pollution. The 95%CI is a statistic used to estimate the parameter p. It indicates that when conducting a large number of independent identical experiments, we can be 95% confident that the true regression coefficient falls within this range. Furthermore, a narrower confidence interval signifies a higher precision of our estimate. In the AST model, the distance to main roads was negatively correlated with AST
Analysis of the correlation between exposure to traffic-related air pollution and other blood indicators
After adjusting for relevant variable factors in the multiple linear models, certain blood indicators of patients with liver cirrhosis showed significant associations with the concentrations of traffic-related pollutants [Table 8]. Specifically, PM10, CO, and O3 had a greater impact on blood indicators, while PM2.5, NO2, and SO2 also affect certain indicators. The exposure concentration of PM2.5 was positively correlated with PT and INR. For every 1 µg/m3 increase in PM2.5 concentration, PT increased by 0.271 [P < 0.05, 95%CI = (0.119, 0.424)], and INR increases by 0.022 [P < 0.05,
Results of regression analysis of traffic-related air pollution and other blood indicators
β (95%CI) | |||||||||
CK | BUN | CHO | TG | PT | INR | SF | Hb | PLT | |
PM2.5 (μg/m3) | -0.02 (-0.13, 0.10) | -0.011 (-0.06, 0.04) | -0.01 (-0.21, 0.19) | -0.02 (-0.07, 0.04) | 0.27 (0.12, 0.43)* | 0.02 (0.00, 0.04)* | 3.31 (-33.35, 39.96) | 1.25 (-1.65, 4.16) | 18.67 (-3.99, 41.33) |
PM10 (μg/m3) | -0.09 (-0.17, -0.01)* | -0.01 (-0.04, 0.03) | -0.07 (-0.22, 0.08) | -0.02 (-0.07, 0.02) | -0.17 (-0.44, 0.11) | -0.029 (-0.054, -0.003)* | -22.90 (-67.25, 21.45) | -2.26 (-4.01, -0.50)* | -16.25 (-31.29, -1.20)* |
SO2 (μg/m3) | 0.16 (-0.04, 0.36) | 0.04 (-0.07, 0.15) | 0.54 (0.13, 0.95)* | -0.02 (-0.14, 0.09) | -0.19 (-1.23, 0.85) | 0.04 (-0.04, 0.12) | -73.98 (-214.12, 66.18) | 1.69 (-4.06, 7.43) | -15.57 (-56.03, 24.89) |
NO2 (μg/m3) | -0.02 (-0.15, 0.11) | 0.06 (-0.01, 0.13) | -0.04 (-0.29, 0.21) | -0.11 (-0.19, -0.03)* | -0.16 (-0.41, 0.09) | 0.00 (-0.03, 0.03) | -27.84 (-75.58, 19.90) | -3.54 (-7.43, 0.36) | -18.73 (-46.88, 9.43) |
CO (mg/m3) | 4.83 (-0.41, 10.06) | -2.28 (-4.44, -0.13)* | 6.52 (-5.24, 18.29) | 3.98 (0.74, 7.22)* | -3.99 (-13.95, 5.97) | -0.34 (-1.75, 1.06) | 354.41 (-2,317.39, 3,026.21) | 165.95 (26.21, 305.70)* | 400.68 (-554.22, 1,355.58) |
O3 (μg/m3) | 0.069 (0.01, 0.13)* | -0.01 (-0.04, 0.03) | 0.13 (0.01, 0.25)* | -0.03 (-0.06, 0.01) | 0.03 (-0.10, 0.15) | 0.01 (0.00, 0.02)* | -13.53 (-33.88, 6.82) | 1.22 (-0.83, 3.27) | -4.73 (-18.52, 9.05) |
Distance from the secondary road (m) | -0.001 (-0.002, 0) | > -0.001 (-0.001, 0.001) | > -0.001 (-0.002, 0.002) | 0.001 (0, 0.002)* | -0.004 (-0.007, -0.001)* | > -0.001 (-0.001, 0) | -0.21 (-0.88, 0.47) | 0.01 (-0.03, 0.05) | 0.04 (-0.18, 0.25) |
Distance from the main road (m) | < 0.001 (-0.001, 0.001) | < 0.001 (0, 0.001) | 0.002 (0, 0.003)* | > -0.001 (-0.001, 0) | 0.001 (0, 0.003)* | < 0.001 (-)* | -0.21 (-0.35, -0.06)* | 0.01 (-0.01, 0.04) | 0.06 (-0.12, 0.23) |
Distance from the highway (km) | 0.01 (-0.08, 0.09) | -0.01 (-0.06, 0.03) | 0.00 (-0.18, 0.18) | -0.01 (-0.06, 0.04) | 0.24 (0.10, 0.38)* | 0.01 (0.00, 0.03) | 6.95 (-18.90, 32.80) | 0.35 (-2.22, 2.91) | 8.10 (-8.24, 24.43) |
There was a certain association between other blood indicators of patients with liver cirrhosis and the level of traffic exposure. The distance to the secondary road was positively correlated with log(TG) and negatively correlated with PT levels. For every 1-meter increase in distance to the secondary road, log(TG) increased by 0.001 [P < 0.05, 95%CI = (0, 0.002)], and PT decreases by 0.004 [P < 0.05, 95%CI = (-0.007, -0.001)]. The distance to the main road was positively correlated with CHO, PT, and INR, and negatively correlated with SF. For every 1-meter increase in distance to the main road, CHO increased by 0.002 [P < 0.05, 95%CI = (0, 0.003)], PT increases by 0.001 [P < 0.05, 95%CI = (0, 0.00)], and SF decreases by 0.205 [P < 0.05, 95%CI =
DISCUSSION
Liver injury assessments typically include measuring ALT, AST, and GGT levels, with ALT and AST being the most commonly used biomarkers for liver injury in clinical diagnosis and research[31]. Within the spectrum of liver enzymes, ALT is predominantly expressed in the cytoplasm of liver cells, exhibiting strong hepatic tissue specificity. Thus, elevated ALT activity reflects the extent of liver cell damage. In contrast, AST is also expressed in tissues outside the liver, resulting in a lower specificity for liver cell injury[32]. Previous studies have shown that ALT is primarily associated with hepatic lipid changes[33], while AST is more closely related to liver inflammation[34]. Multiple cohort studies conducted both domestically and internationally have found a close association between liver enzymes and air pollution related to traffic. For instance, a cohort study evaluating liver toxicity risk among police department employees exposed to urban pollution found significantly increased levels of ALT and AST in the exposed group’s serum[35]. In a prospective cohort study in Taiwan, long-term exposure to PM2.5 was dose-dependently correlated with serum ALT levels and positively associated with subsequent risk of liver cancer during long-term follow-up[36].
In our study, we observed that the correlation between ALT and traffic-related air pollution, as well as traffic exposure distance, was not significant, whereas AST showed a positive correlation with proximity to main roads. This suggests that, in comparison to the impact on hepatic lipid accumulation and pathology, increasing the intensity of traffic exposure may exacerbate inflammation at the hepatic site in patients with cirrhosis.
GGT is closely associated with oxidative stress and serves as an indicator of liver damage. It participates in the breakdown of glutathione (GSH) and is essential for GSH-related biological transformation processes and the detoxification of exogenous substances[37]. In a cross-sectional study involving 150 newborns, maternal exposure to PM was found to be significantly and positively correlated with GGT levels in the umbilical cord blood of newborns. Additionally, the total length of streets within a 100-meter buffer zone around homes was also significantly positively correlated with GGT levels[38]. Two cohort studies in Germany involving 5,892 adults revealed a positive correlation between long-term air pollution and blood GGT concentration, particularly with respect to PM2.5[14]. Similar results by cross-sectional studies were found in Taiwan, showing that long-term exposure to air pollution was associated with elevated levels of GGT[39], which is consistent with the analysis of a nationally representative sample of 32,989 individuals from the Canadian Health Measures Survey[40]. In a large population cohort in Vorarlberg, Austria, exposure to PM and NOx was positively correlated with increased GGT concentration, with transportation contributing significantly to this correlation[37]. This is in line with our finding of a negative correlation trend between blood GGT concentration and distance from main roads.
This study found that the levels of CHO were positively correlated with the exposure concentrations of SO2 and O3, while the levels of TG were positively correlated with the exposure concentration of CO. These results indicate that air pollution may increase the risk of liver metabolic abnormalities and fatty liver in patients, supported by previous research. Epidemiological studies have also demonstrated that exposure to air pollution may lead to increased levels of TG and CHO[41-43], and may even induce metabolic syndrome[44]. Animal experiments have shown that exposure to air pollution can lead to significant inflammatory responses, increased oxidative stress in tissues and systemically, abnormal blood lipids, and enhanced pro-inflammatory state of epididymal fat[45].
To date, there have been no other reports examining the correlation between traffic-related air pollution and other indicators. CHE is a glycoprotein synthesized by liver tissue and is commonly used in clinical practice to assess liver function. Our research indicates that the concentrations of NO2 and CO, and the distance from secondary roads are associated with abnormal fluctuations in CHE levels. Prolonged exposure may further lead to liver function abnormalities, potentially resulting in serious consequences such as liver failure. PT refers to the time required for plasma lacking PLT to convert prothrombin into thrombin when excess tissue thromboplastin and calcium ions are added. This extrinsic coagulation pathway requires the involvement of coagulation factors II, V, VII, and X, all of which are synthesized by the liver. Therefore, PT, to some extent, reflects liver function, reserves, severity of pathological changes, and prognosis. PT levels are positively correlated with exposure concentrations of PM2.5 and negatively correlated with the distance from secondary roads. Secondary roads often face congestion, leading to frequent deceleration and idling of vehicles, resulting in higher emissions of PM2.5 from vehicle exhaust, brake, and tire wear. These may have adverse effects on certain liver function indicators in exposed individuals.
Research on the mechanisms of air pollution-induced liver damage is gradually attracting broad attention in the academic community. Studies have indicated that air pollutants primarily cause harm to health through oxidative stress and inflammatory responses. Prolonged exposure to air pollutants can trigger respiratory inflammation reactions, potentially leading to systemic inflammation[46] and inducing the production of additional reactive oxygen species in organs such as the liver[47]. Additionally, PM2.5 can enter the alveoli and circulate in the bloodstream through the pulmonary system[48]. When pollutants move from the lungs to the liver, they may activate immune cells, prompting the secretion of pro-inflammatory cytokines and creating a pro-inflammatory environment[49,50] conducive to various liver diseases. Moreover, cytochrome P-450 enzymes are the major producers of reactive oxygen species and are widely present in the liver, further increasing the risk of liver damage[51]. Traffic-related air pollution includes diesel exhaust particles (DEP) emitted by diesel engine vehicles. DEP can generate reactive oxygen species through non-enzymatic processes or enzyme-catalyzed reactions mediated by cytochrome P-450, leading to a certain degree of liver damage due to excessive oxidative stress[17]. Research on the mechanisms of health effects of traffic-related air pollution also provides a basis and new insights for related epidemiological studies.
In this study, we found a significant correlation between TRAP and changes in blood biomarkers among patients with liver cirrhosis. Drawing on previous research, it can be inferred that the liver structure and function of these patients are compromised to varying degrees[52], making them more sensitive to exposure to TRAP. Such exposure may lead to increased oxidative stress[5,20] throughout the body, especially in the liver, and result in metabolic dysfunction[21,22]. This situation can exacerbate liver inflammation[24], and prolonged exposure may contribute to the development of fatty liver[26] and further deterioration of liver function[27]. Consequently, this could negatively affect the treatment and recovery of patients in the decompensated phase of liver cirrhosis.
Limitations and prospects
There are also some limitations in this study. Firstly, due to the incomplete recording of address information for many patients in the study cohort, the sample size was insufficient to use a proximity model to fully elucidate the effect of traffic-related air pollution on blood indicators in patients with cirrhosis. Secondly, the study results may have been influenced by various confounding factors, leading to some degree of error in certain results. For example, regular exercise has been shown to affect individual antioxidant activity and baseline oxidative stress, thereby reducing liver damage. Future research could enhance the accuracy of results by further adjusting the model.
As the global energy transition progresses, competition between traditional fuel-powered vehicles and electric vehicles is intensifying. Against the backdrop of growing environmental awareness, technological advancements, and policy initiatives, electric vehicles are gradually gaining favor in the market[53]. Future research will separately investigate the health effects of traffic pollution caused by traditional fuel-powered vehicles and electric vehicles, thereby enhancing the practical significance and academic value of the studies.
Conclusions
Liver cirrhosis represents a significant global health burden[54], accounting for approximately 1.27 million deaths and 38.9 million DALYs lost each year[55]. In China, the number of patients is estimated to reach 7 million, far surpassing the figures in developed countries such as Europe and North America[56]. This study focuses on patients with liver cirrhosis and specifically examines the impact of traffic-related air pollution on their health. Our findings indicate that exposure to high levels of traffic pollution has a significantly adverse effect on liver function indicators. This may impede liver metabolism, exacerbate oxidative stress, promote liver injury, and increase the risk of various liver diseases. Furthermore, it could indirectly influence the occurrence of liver decompensation events. Therefore, addressing and improving traffic-related air pollution is crucial for protecting liver function and enhancing the health of patients with liver cirrhosis. From an individual perspective, patients should try to choose residences that are away from busy traffic areas, use air purifiers, wear masks, and maintain a healthy diet and regular health check-ups to reduce the risk of exposure to traffic pollution. From a governmental perspective, urban planning should be optimized to minimize the proximity of major traffic thoroughfares to densely populated areas. Additionally, promoting clean transportation, strictly enforcing vehicle emission standards, increasing urban green spaces, and conducting health education are essential steps to effectively reduce the impact of traffic pollution on public health, especially for patients with liver cirrhosis.
DECLARATIONS
Authors’ contributions
Manuscript writing, revision, visualization, and formal analysis: Hu Q
Conceptualization, methodology, investigation, and data curation: Zhao L
Data reduction and analysis: Wang Z
Data curation and editing: Han P, Ding J
Funding acquisition, project administration, and resources: Wang T, Li J
Supervision and resources: Mao H
Availability of data and materials
The datasets generated during the current study are available from the corresponding author upon reasonable request.
Financial support and sponsorship
This study is supported by the Fundamental Research Funds for the Central Universities of China [63241318, 63241322] and Tianjin Key Medical Discipline (Specialty) Construction Project (TJYXZDXK - 059B).
Conflicts of interest
Mao H is an Editorial Board member of Journal of Environmental Exposure Assessment. Mao H was not involved in any steps of editorial processing, notably including reviewers’ selection, manuscript handling, and decision making. The other authors declared that there are no conflicts of interest.
Ethical approval and consent to participate
This study has been approved by the Ethics Committee of Second People’s Hospital of Tianjin ([2015]15). All participants signed a written informed consent document prior to enrollment.
Consent for publication
Not applicable.
Copyright
© The Author(s) 2024.
REFERENCES
1. Guan WJ, Zheng XY, Chung KF, Zhong NS. Impact of air pollution on the burden of chronic respiratory diseases in China: time for urgent action. Lancet. 2016;388:1939-51.
2. Shaddick G, Thomas ML, Mudu P, Ruggeri G, Gumy S. Half the world’s population are exposed to increasing air pollution. npj Clim Atmos Sci. 2020;3:124.
3. Bai X, Chen H, Oliver BG. The health effects of traffic-related air pollution: a review focused the health effects of going green. Chemosphere. 2022;289:133082.
4. GBD 2021 Risk Factors Collaborators. Global burden and strength of evidence for 88 risk factors in 204 countries and 811 subnational locations, 1990-2021: a systematic analysis for the Global Burden of Disease Study 2021. Lancet. 2024;403:2162-203.
5. Costa LG, Cole TB, Coburn J, Chang YC, Dao K, Roqué PJ. Neurotoxicity of traffic-related air pollution. Neurotoxicology. 2017;59:133-9.
6. Harrison RM, Allan J, Carruthers D, et al. Non-exhaust vehicle emissions of particulate matter and VOC from road traffic: a review. Atmos Environ. 2021;262:118592.
7. Haddad P, Kutlar Joss M, Weuve J, et al. Long-term exposure to traffic-related air pollution and stroke: a systematic review and meta-analysis. Int J Hyg Environ Health. 2023;247:114079.
8. Aryal A, Harmon AC, Dugas TR. Particulate matter air pollutants and cardiovascular disease: strategies for intervention. Pharmacol Ther. 2021;223:107890.
10. Xing YF, Xu YH, Shi MH, Lian YX. The impact of PM2.5 on the human respiratory system. J Thorac Dis. 2016;8:E69-74.
11. Cacciottolo M, Wang X, Driscoll I, et al. Particulate air pollutants, APOE alleles and their contributions to cognitive impairment in older women and to amyloidogenesis in experimental models. Transl Psychiat. 2017;7:e1022.
12. Chen H, Kwong JC, Copes R, et al. Living near major roads and the incidence of dementia, Parkinson’s disease, and multiple sclerosis: a population-based cohort study. Lancet. 2017;389:718-26.
13. Mann JK, Lutzker L, Holm SM, et al. Traffic-related air pollution is associated with glucose dysregulation, blood pressure, and oxidative stress in children. Environ Res. 2021;195:110870.
14. Markevych I, Wolf K, Hampel R, et al. Air pollution and liver enzymes. Epidemiology. 2013;24:934-5.
15. Qiu W, Zhou Y, He H, et al. Short-term effects of air pollution on liver function among urban adults in China. Atmos Environ. 2021;245:118011.
16. Kim HJ, Min JY, Seo YS, Min KB. Association of ambient air pollution with increased liver enzymes in Korean adults. Int J Environ Res Public Health. 2019;16:1213.
17. Kim KN, Lee H, Kim JH, Jung K, Lim YH, Hong YC. Physical activity- and alcohol-dependent association between air pollution exposure and elevated liver enzyme levels: an elderly panel study. J Prev Med Public Health. 2015;48:151-69.
18. Tan C, Wang Y, Lin M, et al. Long-term high air pollution exposure induced metabolic adaptations in traffic policemen. Environ Toxicol Pharmacol. 2018;58:156-62.
19. Li Q, Liu H, Alattar M, et al. The preferential accumulation of heavy metals in different tissues following frequent respiratory exposure to PM2.5 in rats. Sci Rep. 2015;5:16936.
20. Brook RD, Brook JR, Rajagopalan S. Air pollution: the “Heart” of the problem. Curr Hypertens Rep. 2003;5:32-9.
21. Yu Y, Paul K, Arah OA, et al. Air pollution, noise exposure, and metabolic syndrome - a cohort study in elderly Mexican-Americans in Sacramento area. Environ Int. 2020;134:105269.
22. Matthiessen C, Lucht S, Hennig F, et al; Heinz Nixdorf Recall Study Investigative Group. Long-term exposure to airborne particulate matter and NO2 and prevalent and incident metabolic syndrome - results from the Heinz Nixdorf Recall Study. Environ Int 2018;116:74-82.
23. Voss S, Schneider A, Huth C, et al. ENVINT-D-20-01309: long-term exposure to air pollution, road traffic noise, residential greenness, and prevalent and incident metabolic syndrome: results from the population-based KORA F4/FF4 cohort in Augsburg, Germany. Environ Int. 2021;147:106364.
24. Chen J, Wu L, Yang G, et al. The influence of PM2.5 exposure on non-alcoholic fatty liver disease. Life Sci. 2021;270:119135.
25. Sun Q, Yue P, Deiuliis JA, et al. Ambient air pollution exaggerates adipose inflammation and insulin resistance in a mouse model of diet-induced obesity. Circulation. 2009;119:538-46.
26. Qiu YN, Wang GH, Zhou F, et al. PM2.5 induces liver fibrosis via triggering ROS-mediated mitophagy. Ecotoxicol Environ Saf. 2019;167:178-87.
27. Pedersen M, Andersen ZJ, Stafoggia M, et al. Ambient air pollution and primary liver cancer incidence in four European cohorts within the ESCAPE project. Environ Res. 2017;154:226-33.
28. Zheng Z, Xu X, Zhang X, et al. Exposure to ambient particulate matter induces a NASH-like phenotype and impairs hepatic glucose metabolism in an animal model. J Hepatol. 2013;58:148-54.
29. Laing S, Wang G, Briazova T, et al. Airborne particulate matter selectively activates endoplasmic reticulum stress response in the lung and liver tissues. Am J Physiol Cell Physiol. 2010;299:C736-49.
30. Dybdahl M, Risom L, Møller P, et al. DNA adduct formation and oxidative stress in colon and liver of Big Blue rats after dietary exposure to diesel particles. Carcinogenesis. 2003;24:1759-66.
31. McGill MR. The past and present of serum aminotransferases and the future of liver injury biomarkers. EXCLI J. 2016;15:817-28.
32. Lioudaki E, Ganotakis ES, Mikhailidis DP. Liver enzymes: potential cardiovascular risk markers? Curr Pharm Des. 2011;17:3632-43.
33. Westerbacka J, Cornér A, Tiikkainen M, et al. Women and men have similar amounts of liver and intra-abdominal fat, despite more subcutaneous fat in women: implications for sex differences in markers of cardiovascular risk. Diabetologia. 2004;47:1360-9.
34. Baou K, Vlachopoulos C, Manesis E, Archimandritis A, Stefanadis C. Non-alcoholic fatty liver and cardiovascular disease: an emerging relationship. Hellenic J Cardiol. 2007;48:37-41.
35. Tomao E, Baccolo TP, Sacchi L, DE Sio S, Tomei F. Harm to the liver among employees of the Municipal Police Force. Int J Environ Health Res. 2002;12:145-51.
36. Pan WC, Wu CD, Chen MJ, et al. Fine particle pollution, alanine transaminase, and liver cancer: a Taiwanese prospective cohort study (REVEAL-HBV). J Natl Cancer Inst. 2016;108:djv341.
37. Wirsching J, Nagel G, Tsai MY, et al. Exposure to ambient air pollution and elevated blood levels of gamma-glutamyl transferase in a large Austrian cohort. Sci Total Environ. 2023;883:163658.
38. Pejhan A, Agah J, Adli A, et al. Exposure to air pollution during pregnancy and newborn liver function. Chemosphere. 2019;226:447-53.
39. Zhang Z, Guo C, Chang LY, et al. Long-term exposure to ambient fine particulate matter and liver enzymes in adults: a cross-sectional study in Taiwan. Occup Environ Med. 2019;76:488-94.
40. Dales R, Mitchell K, Lukina A, Brook J, Karthikeyan S, Cakmak S. Does ambient air pollution influence biochemical markers of liver injury? Findings of a cross-sectional population-based survey. Chemosphere. 2023;340:139859.
41. Montes JOA, Villarreal AB, Piña BGB, et al. Short-term ambient air ozone exposure and components of metabolic syndrome in a cohort of mexican obese adolescents. Int J Environ Res Public Health. 2023;20:4495.
42. Vander Hoorn S, Murray K, Nedkoff L, et al. Long-term exposure to outdoor air pollution and risk factors for cardiovascular disease within a cohort of older men in Perth. PLoS One. 2021;16:e0248931.
43. Tomao E, Tiziana PB, Rosati V, Marcellini L, Tomei F. The effects of air pollution on the lipid balance of traffic police personnel. Ann Saudi Med. 2002;22:287-90.
44. Yang BY, Qian ZM, Li S, et al. Long-term exposure to ambient air pollution (including PM1) and metabolic syndrome: the 33 Communities Chinese Health Study (33CCHS). Environ Res. 2018;164:204-11.
45. Wei Y, Zhang JJ, Li Z, et al. Chronic exposure to air pollution particles increases the risk of obesity and metabolic syndrome: findings from a natural experiment in Beijing. FASEB J. 2016;30:2115-22.
46. Robinson MW, Harmon C, O’Farrelly C. Liver immunology and its role in inflammation and homeostasis. Cell Mol Immunol. 2016;13:267-76.
47. Romieu I, Castro-Giner F, Kunzli N, Sunyer J. Air pollution, oxidative stress and dietary supplementation: a review. Eur Respir J. 2008;31:179-97.
48. Zhao J, Gao Z, Tian Z, et al. The biological effects of individual-level PM(2.5) exposure on systemic immunity and inflammatory response in traffic policemen. Occup Environ Med. 2013;70:426-31.
49. Kim JW, Park S, Lim CW, Lee K, Kim B. The role of air pollutants in initiating liver disease. Toxicol Res. 2014;30:65-70.
50. Tan HH, Fiel MI, Sun Q, et al. Kupffer cell activation by ambient air particulate matter exposure may exacerbate non-alcoholic fatty liver disease. J Immunotoxicol. 2009;6:266-75.
51. Tomaru M, Takano H, Inoue K, et al. Pulmonary exposure to diesel exhaust particles enhances fatty change of the liver in obese diabetic mice. Int J Mol Med. 2007;19:17-22.
52. Ginès P, Krag A, Abraldes JG, Solà E, Fabrellas N, Kamath PS. Liver cirrhosis. Lancet. 2021;398:1359-76.
53. GBD 2017 Cirrhosis Collaborators. The global, regional, and national burden of cirrhosis by cause in 195 countries and territories, 1990-2017: a systematic analysis for the Global Burden of Disease Study 2017. Lancet Gastroenterol Hepatol. 2020;5:245-66.
54. Devarbhavi H, Asrani SK, Arab JP, Nartey YA, Pose E, Kamath PS. Global burden of liver disease: 2023 update. J Hepatol. 2023;79:516-37.
55. Xiao J, Wang F, Wong NK, et al. Global liver disease burdens and research trends: analysis from a Chinese perspective. J Hepatol. 2019;71:212-21.
Cite This Article
How to Cite
Download Citation
Export Citation File:
Type of Import
Tips on Downloading Citation
Citation Manager File Format
Type of Import
Direct Import: When the Direct Import option is selected (the default state), a dialogue box will give you the option to Save or Open the downloaded citation data. Choosing Open will either launch your citation manager or give you a choice of applications with which to use the metadata. The Save option saves the file locally for later use.
Indirect Import: When the Indirect Import option is selected, the metadata is displayed and may be copied and pasted as needed.
About This Article
Copyright
Data & Comments
Data
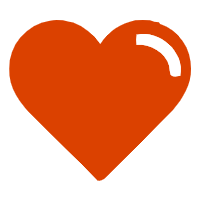
Comments
Comments must be written in English. Spam, offensive content, impersonation, and private information will not be permitted. If any comment is reported and identified as inappropriate content by OAE staff, the comment will be removed without notice. If you have any queries or need any help, please contact us at support@oaepublish.com.