Mesenchymal stromal cells-extracellular vesicles: protein corona as a camouflage mechanism?
Abstract
Mesenchymal stromal cells (MSCs) showed promising potential for regenerative and therapeutic applications for several pathologies and conditions. Their potential is mainly ascribed to the factors and extracellular vesicles (EVs) they release, which are now envisioned as cell-free therapeutics in cutting-edge clinical studies. A main cornerstone is the preferential uptake by target cells and tissues, in contrast to clearance by phagocytic cells or removal from circulation before reaching the final destination. Recent literature has suggested how the surface properties of EVs might influence their half-life, bio-distribution, and specific uptake. In particular, the concept of a protein corona surrounding EVs emerged. Especially for culture-purified EVs, the process of tailoring a treatment or tissue-specific corona was explored. Liam-Or et al. examined the impact of protein corona on MSC-EVs when specific proteins, preferentially albumin, were adsorbed from media on the EV surface before isolation. This resulted in improved uptake by liver parenchymal cells and reduced incorporation by macrophages, together with an increased half-life in the circulation system. Thus, producing MSC-EVs with an albumin-enriched protein corona might be a camouflage strategy to enhance non-phagocytic uptake in the liver. This research might be a milestone for future studies on other EVs-camouflage approaches tailored to specific tissues and therapeutic applications.
Keywords
MAIN TEXT
Mesenchymal stromal cells (MSCs) were first identified in the bone marrow in the late 1960s by Friedenstein et al. as multipotent cells able to differentiate into osteoblasts, adipocytes, and chondrocytes in vitro[1]. In recent years, it became clear that similar cell types might be identified in several other tissues[2], including adipose tissue, placenta, umbilical cord, and umbilical cord blood. The first detailed characterization of MSCs was performed by Caplan[3] in the 1990s, who proposed in the 2010s the nowadays most accepted concept of “Medicinal Signalling Cells”[4], due to their ability to secrete bioactive factors (the secretome) that are immunomodulatory and trophic (regenerative)[5]. Therefore, in the clinical setting, MSCs are now believed to stimulate the patient’s own site-specific and tissue-specific resident stem cells, rather than differentiate into the damaged tissues; additionally, MSCs also modulate the immune response[6]. As of October 2024, almost 1,700 clinical trials are registered (https://clinicaltrials.gov/, search term: Mesenchymal Stem/Stromal Cells), evaluating the effectiveness of MSCs in treating, inter alia, orthopedic conditions, cardiovascular diseases, graft-versus-host disease (GvHD), and brain and neurological disorders, with some studies also focusing on immune system diseases.
Due to its properties resembling those of secreting cells, the interest in MSC-derived secretome is notably increasing, with nearly 30 registered clinical trials investigating its potential for osteoarthritis, cerebrovascular and neurological pathologies, and respiratory diseases, including COVID-19 (https://clinicaltrials.gov/, search term: Mesenchymal Stem/Stromal Cells Secretome). The secretome[6] consists of both soluble factors (growth factors, cytokines, chemokines and hormones) and lipid nanoparticles like extracellular vesicles (EVs). EVs are classified into small EVs (sEVs, < 200 nm, also improperly called “exosomes”) or large EVs (lEVs, > 200 nm) depending on their size according to Minimal Information for EVs 2023[7]. Due to recent evidence that MSC-EVs have an important role in mediating the regenerative and immunomodulatory properties of MSCs[8], purified EVs are also currently tested in almost 50 clinical studies (https://clinicaltrials.gov/, search term: Mesenchymal Stem/Stromal Cells EVs/Exosomes). EVs shuttle several molecules, such as proteins, lipids and nucleic acids, including miRNAs[9]. In particular, miRNAs were shown to be involved in regenerative and immune-related processes[10], such as osteoarthritis[11], neurological[12] and cardiovascular diseases[13], and the immune system[14]. Despite this increasing knowledge in EV cargo, an underestimated issue is the molecular characterization of how EVs interact with and are taken up by target cells and tissues. EVs binding is regulated by a complex process that involves several factors, including the composition of the membranes, such as protein, lipid, and glycan. As an example, tetraspanins in EVs play an essential role interacting with integrins and adhesion receptors[15], as well as lipid membrane components, like phosphatidylserine, do with target cell membrane receptors[16], and proteoglycans with lectins[17].
Recently, together with proteins, lipids, and glycans that define the bilayer architecture of EVs, other molecules have been described as involved with EV interaction and targeting. A set of proteins and other biological compounds have been demonstrated to be adsorbed around EVs and constitute an external layer named corona[18]. Two distinct layers may be described: inner (hard) and outer (soft). Irreversible absorption of proteins around EVs leads to the formation of the inner hard layer, while reversible interaction of low-affinity proteins results in the formation of the outer soft layer[19]. Very little knowledge is present regarding the composition of soft and hard layers, although the formation of the corona around EVs inside aqueous phases was postulated to happen via electrostatic interactions and protein aggregation in an environment-dependent fashion[20]. As an example, for plasma EVs, there is a high overlap in the composition of their protein corona with plasma protein aggregates[20] that may correlate with the surface of EVs. Accordingly, a corona layer was found on human-platelet-lysate-derived EVs, which exhibited regenerative and immunomodulatory properties on T cells in vitro[21]. For culture-derived EVs, such as MSC-EVs, a two-step process may occur. During production in cell culture, a first layer can be assembled, and after injection, the interaction with biological fluids may lead to the formation of an environment-dependent layer. Therefore, both a “general” layer dependent on the production process and a “specific” layer related to the injection route have to be studied for clinical translation. Consistently, a corona containing proangiogenic factors from placental expanded MSCs was found on their EVs, and this corona played a key role in mediating vascularized skin regeneration in vivo[22]. However, the relative contribution of these corona layers to particle properties and in vivo behavior has not been thoroughly investigated to date.
A recent study by Liam-Or et al. studied how culturing methods affect MSC-EVs corona compositions and impact accumulation in liver cells and how targeting is dependent on key proteins that mediate the in vivo behavior of EVs[23]. EVs were produced from umbilical cord MSCs (ucMSCs) in both 2-dimensional and 3-dimensional cultures. Serum-free basal medium and 20% KnockOut serum replacement (KO)-containing basal medium were used for 2D and 3D MSC cultures, respectively. EVs were purified with a sucrose gradient centrifugation before a final wash step and stored at -80 °C[23]. Although having a similar marker expression, zeta-potential values and size, EV3D had a three-fold higher protein content than EV2D. Consistently, distinct protein profiles between EV2D and EV3D can be observed, with EV3D showing similarities to KO medium proteins which were absent in EV2D[23]. This was confirmed by mass spectrometry, with serum albumin being among the most abundant proteins in both EV3D and KO supplements, forming the inner corona during culture. On the contrary, EV2D cultured in basal medium without supplements were covered with MSC secretome proteins[23]. Additionally, exposing EVs to EVs-depleted FBS (fetal bovine serum) allowed for the formation of an outer corona with different preferential compositions when comparing 2D and 3D (EV with bound hard corona: HC-EV2D and HC-EV3D). For example, bovine serum albumin was again more abundant in EV3D with respect to EV2D. Thus, culture medium (pure basal or KO-supplemented) and subsequent FBS incubation allowed for the formation of specific layers, termed the primary and secondary corona, respectively[23].
The authors further explored the role of different corona in EV uptake using both in vitro and in vivo systems. In the in vitro study, they characterized the uptake of EVs by macrophages and liver parenchymal cells, focusing on strategies to enhance EV accumulation in liver cells other than in phagocytic cells. The presence of the corona increased the uptake in both cell types for EV2D, while EV3D exhibited a significant reduction in uptake by phagocytic cells and a corresponding increase in uptake by parenchymal cells[23]. Overall, 3D-cultured EVs were preferentially internalized by parenchymal cells, and their reduced uptake by phagocytic cells indicated a more favorable interaction with the liver microenvironment
Eventually, authors proposed that albumin on the inner corona may attract more albumin to form the outer layer, and therefore, increased uptake observed for albumin-coated EV3D might be dependent on albumin receptors and their subtype on target cells and tissues. The first hypothesis was confirmed by producing EV2D in the presence of exogenous albumin, which enabled the formation of an albumin-enriched inner layer that further attracted albumin[23] for the second layer. The second hypothesis was endorsed in experiments in which blocking albumin receptors reduced liver uptake of both EV3D and EV2D produced in the presence of albumin. Of note, the characteristics of EV2D were observed only when vesicles were generated in the presence of albumin, not when particles were supplemented after their isolation[23]. This implies that the formation of the first corona at the site of secretion is essential.
These results showed how albumin coating is a requisite for the efficient uptake of EVs by hepatic cells and how it may prolong the half-life of EVs. Supporting this, a recent study demonstrated that an albumin-dominated corona formed on lipid-like nanoparticles preferentially binds to receptors expressed on primary hepatocytes[24]. Additionally, EVs engineered to bind albumin exhibit extended circulation times, resulting in prolonged half-life[25]. Further research is needed to understand the possibility to prolong the blood-circulation time of albumin-enriched corona on EVs, a crucial requisite for prolonged therapeutic activity that may also depend on other key factors.
In this frame, the choice between 2D and 3D cell culture is a crucial step. In 2D cultures, cells grow on flat surfaces, often leading to altered cell morphology and less natural protein expression. This artificial environment may produce EVs with atypical protein coronas that do not accurately represent in vivo conditions, potentially limiting their camouflage efficacy in biological systems[26]. In contrast, 3D cultures better mimic in vivo environments by facilitating complex cell-cell and cell-matrix interactions, leading to more physiologically relevant EVs with protein coronas closer to those found in natural tissue. These EVs may display enhanced immune evasion and targeted delivery capabilities[27]. However, 3D cultures present challenges, including higher complexity, longer culture times, and increased cost. Additionally, the heterogeneity within 3D systems can make EV characterization difficult, as it may result in diverse EV subpopulations with varying protein coronas[28]. Ultimately, while 3D cultures offer a more accurate reflection of in vivo EV protein composition and camouflage potential, they are more demanding to manage and analyze.
Overall, the “protein corona-extracellular vesicle complex” represents a promising approach in EV-based therapeutic delivery, particularly for improved cell targeting and immune evasion. The protein corona significantly alters the interactions of EVs with cells and immune components. Understanding and manipulating this complex offers exciting opportunities but also poses several challenges. A key challenge is the lack of control over the composition of the protein corona, which can vary depending on the individual’s physiology and disease state, thus impacting targeting efficiency and immune evasion. Additionally, protein corona formation can mask EV surface proteins critical for binding to target cells, reducing therapeutic specificity. Further research is needed to develop methods for controlling corona composition, perhaps by engineering EV surfaces to selectively recruit beneficial proteins for immune evasion and cellular targeting[18]. Moreover, developing standard protocols for corona-EV complex characterization is essential for clinical translation, as these parameters affect biodistribution and therapeutic efficacy[29]. As insights into corona-EV interactions deepen, synthetic corona layers and tailored EV engineering may enable more precise, patient-specific treatments with fewer immunogenic risks.
In conclusion, Liam-Or et al. confirmed the contribution of corona enriched with albumin in prolonging the half-life of EVs and enhancing their uptake in liver parenchymal cells, particularly in relation to macrophages[23]. Future research will be crucial to tailor EV features for clinical applications, particularly those derived from MSCs, which have shown promise as therapeutic candidates for various diseases and pathologies.
DECLARATIONS
Authors’ contributions
Conceptualized and wrote the manuscript: Ragni E, Taiana M
Availability of data and materials
Raw data are available at: https://osf.io/pd9tb/?view_only=27ca5142990444aeb903eb86fe5748e1.
Financial support and sponsorship
This work was supported and funded by the Italian Ministry of Health (Ricerca Corrente).
Conflicts of interest
Ragni E is a Junior Editorial Board member of the journal Extracellular Vesicles and Circulating Nucleic Acids. Taiana M declared that there are no conflicts of interest.
Ethical approval and consent to participate
Not applicable.
Consent for publication
Not applicable.
Copyright
© The Authors 2024.
REFERENCES
1. Friedenstein AJ, Chailakhjan RK, Lalykina KS. The development of fibroblast colonies in monolayer cultures of guinea-pig bone marrow and spleen cells. Cell Tissue Kinet 1970;3:393-403.
2. Hass R, Kasper C, Böhm S, Jacobs R. Different populations and sources of human mesenchymal stem cells (MSC): a comparison of adult and neonatal tissue-derived MSC. Cell Commun Signal 2011;9:12.
4. Caplan AI. Mesenchymal stem cells: time to change the name! Stem Cells Transl Med 2017;6:1445-51.
6. Trigo CM, Rodrigues JS, Camões SP, Solá S, Miranda JP. Mesenchymal stem cell secretome for regenerative medicine: where do we stand? J Adv Res 2024;Online ahead of print.
7. Welsh JA, Goberdhan DCI, O'Driscoll L, et al; MISEV Consortium. Minimal information for studies of extracellular vesicles (MISEV2023): from basic to advanced approaches. J Extracell Vesicles 2024;13:e12404.
8. Kou M, Huang L, Yang J, et al. Mesenchymal stem cell-derived extracellular vesicles for immunomodulation and regeneration: a next generation therapeutic tool? Cell Death Dis 2022;13:580.
9. Doyle LM, Wang MZ. Overview of extracellular vesicles, their origin, composition, purpose, and methods for exosome isolation and analysis. Cells 2019;8:727.
10. Li S, Lv D, Yang H, Lu Y, Jia Y. A review on the current literature regarding the value of exosome miRNAs in various diseases. Ann Med 2023;55:2232993.
11. Shang X, Fang Y, Xin W, You H. The application of extracellular vesicles mediated miRNAs in osteoarthritis: current knowledge and perspective. J Inflamm Res 2022;15:2583-99.
12. He M, Zhang HN, Tang ZC, Gao SG. Diagnostic and therapeutic potential of exosomal microRNAs for neurodegenerative diseases. Neural Plast 2021;2021:8884642.
13. Zheng D, Huo M, Li B, et al. The role of exosomes and exosomal microRNA in cardiovascular disease. Front Cell Dev Biol 2020;8:616161.
14. Bauer KM, Round JL, O'Connell RM. No small matter: emerging roles for exosomal miRNAs in the immune system. FEBS J 2022;289:4021-37.
15. Andreu Z, Yáñez-Mó M. Tetraspanins in extracellular vesicle formation and function. Front Immunol 2014;5:442.
16. Graham DK, DeRyckere D, Davies KD, Earp HS. The TAM family: phosphatidylserine sensing receptor tyrosine kinases gone awry in cancer. Nat Rev Cancer 2014;14:769-85.
17. Atai NA, Balaj L, van Veen H, et al. Heparin blocks transfer of extracellular vesicles between donor and recipient cells. J Neurooncol 2013;115:343-51.
18. Heidarzadeh M, Zarebkohan A, Rahbarghazi R, Sokullu E. Protein corona and exosomes: new challenges and prospects. Cell Commun Signal 2023;21:64.
19. Ke PC, Lin S, Parak WJ, Davis TP, Caruso F. A decade of the protein corona. ACS Nano 2017;11:11773-6.
20. Tóth EÁ, Turiák L, Visnovitz T, et al. Formation of a protein corona on the surface of extracellular vesicles in blood plasma. J Extracell Vesicles 2021;10:e12140.
21. Gomes FG, Andrade AC, Wolf M, et al. Synergy of human platelet-derived extracellular vesicles with secretome proteins promotes regenerative functions. Biomedicines 2022;10:238.
22. Wolf M, Poupardin RW, Ebner-Peking P, et al. A functional corona around extracellular vesicles enhances angiogenesis, skin regeneration and immunomodulation. J Extracell Vesicles 2022;11:e12207.
23. Liam-Or R, Faruqu FN, Walters A, et al. Cellular uptake and in vivo distribution of mesenchymal-stem-cell-derived extracellular vesicles are protein corona dependent. Nat Nanotechnol 2024;19:846-55.
24. Miao L, Lin J, Huang Y, et al. Synergistic lipid compositions for albumin receptor mediated delivery of mRNA to the liver. Nat Commun 2020;11:2424.
25. Liang X, Niu Z, Galli V, et al. Extracellular vesicles engineered to bind albumin demonstrate extended circulation time and lymph node accumulation in mouse models. J Extracell Vesicles 2022;11:e12248.
26. Gudbergsson JM, Johnsen KB, Skov MN, Duroux M. Systematic review of factors influencing extracellular vesicle yield from cell cultures. Cytotechnology 2016;68:579-92.
27. Ester M, Day RM. Production and utility of extracellular vesicles with 3D culture methods. Pharmaceutics 2023;15:663.
28. Almeria C, Kreß S, Weber V, Egger D, Kasper C. Heterogeneity of mesenchymal stem cell-derived extracellular vesicles is highly impacted by the tissue/cell source and culture conditions. Cell Biosci 2022;12:51.
Cite This Article
How to Cite
Ragni, E.; Taiana, M. Mesenchymal stromal cells-extracellular vesicles: protein corona as a camouflage mechanism?. Extracell. Vesicles. Circ. Nucleic. Acids. 2024, 5, 722-7. http://dx.doi.org/10.20517/evcna.2024.78
Download Citation
Export Citation File:
Type of Import
Tips on Downloading Citation
Citation Manager File Format
Type of Import
Direct Import: When the Direct Import option is selected (the default state), a dialogue box will give you the option to Save or Open the downloaded citation data. Choosing Open will either launch your citation manager or give you a choice of applications with which to use the metadata. The Save option saves the file locally for later use.
Indirect Import: When the Indirect Import option is selected, the metadata is displayed and may be copied and pasted as needed.
About This Article
Copyright
Data & Comments
Data
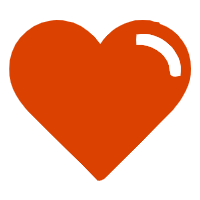
Comments
Comments must be written in English. Spam, offensive content, impersonation, and private information will not be permitted. If any comment is reported and identified as inappropriate content by OAE staff, the comment will be removed without notice. If you have any queries or need any help, please contact us at support@oaepublish.com.