Advanced in-situ/operando characterization techniques: aiding the development of zinc-air batteries
Keywords
Zinc-air batteries (ZABs) are emerging as a frontrunner in next-generation energy storage technology thanks to their high energy density and environmentally friendly attributes. This article explores the critical components of ZABs and highlights recent advances to improve their performance through in-situ/operando studies[1,2].
ZABs are mainly composed of three parts: anodes (usually made of zinc), cathodes (reacting with oxygen), and electrolytes (usually potassium hydroxide solution). This type of battery is considered an up-and-coming energy storage technology due to its high energy density and environmental characteristics. At the cathode, oxygen is reduced through catalytic reactions, while zinc undergoes oxidation reactions at the anode. Typical working conditions include specific temperature and humidity ranges. In addition, factors such as airflow and electrolyte composition can also affect the performance of batteries[3,4].
The cathode in ZABs employs bifunctional catalysts to facilitate both the oxygen reduction reaction (ORR) and the oxygen evolution reaction (OER)[5,6]. An effective catalyst should demonstrate excellent catalytic activity, maintain stability throughout charge and discharge cycles, and ensure high selectivity to prevent the creation of undesirable by-products. Typical catalysts include carbon-based catalysts (such as nitrogen or phosphorus-doped graphite and mesoporous nanocarbons)[7], metal-based catalysts (such as Pt/IrO2, MnO2, and Co9S8/MnS)[8], and composite catalysts (such as metal-carbon nanotube composites and metal oxide-supported nanoparticles)[9]. Developing and optimizing these catalysts are essential for enhancing the performance of ZABs.
ZABs typically use a KOH solution for the electrolyte due to its ease of preparation and high conductivity. However, high-concentration alkaline solutions are prone to volatilization and absorption of CO2, leading to electrolyte degradation[10]. Additionally, the absorption of CO2 can further deteriorate the electrolyte. The potassium hydroxide solution also undergoes a hydrogen evolution reaction (HER). As shown in Figure 1, without considering overpotential, the potential required for hydrogen evolution from water is higher than that needed for zinc deposition across all pH levels. Adding additives such as triethanolamine (TEA) or sodium dodecyl benzene sulfonate (SDBS) can effectively suppress the passivation and deformation of the zinc-anode electrode, enhancing battery performance. Moreover, alternative approaches, including neutral solutions, room-temperature ionic liquids, and solid electrolytes, have been investigated to enhance battery lifespan and improve stability.
Figure 1. Pourbaix diagram for various pH values in water for zinc[11].
Innovative separator design is also a crucial strategy for enhancing the performance of ZABs. The primary purpose of the separator is to prevent direct contact between the cathode and anode. This serves to avoid short circuits, enhance moisture retention, restrict the migration of zincate ions, and inhibit dendrite growth. An ideal separator should possess high mechanical strength, a wide electrochemical window, and strong alkali resistance. By incorporating new materials such as nanofiber-reinforced composite membranes or functionalized polyvinylidene fluoride (PVDF) nanofiber membranes, the performance of the separator can be significantly improved, leading to enhanced overall performance and stability of the battery.
In-situ and operando characterization techniques offer powerful tools for gaining a deeper understanding of the chemical processes in ZABs. As shown in Figure 2, X-ray computed tomography (XCT) is a non-destructive, three-dimensional imaging technique that provides structural information on zinc dendrites and reveals their growth behavior at different current densities. Compared to electron microscopy or Raman imaging, XCT offers higher spatial resolution with minimal damage to the sample. Optical microscopy imaging (OMI), the simplest and most cost-effective observation method, effectively captures phenomena such as growth and volume expansion of zinc dendrites. Transmission electron microscopy (TEM) uses high-energy electron imaging to achieve a high resolution of 0.1 nanometers, making it ideal for observing changes in sample morphology and catalyst aggregation. Magnetic resonance imaging (MRI) detects the motion of atomic nuclei in a magnetic field, allowing for non-destructive, rapid imaging, making it suitable for observing electrolyte and ion concentration distributions. As shown in Figure 3, Raman spectroscopy provides chemical information by detecting the Raman scattering light emitted by the sample. Due to the small Raman scattering cross-section of water, its signal is weak, making Raman spectroscopy particularly suitable for substance analysis in aqueous solutions. X-ray diffraction (XRD) is a non-destructive method for detecting structural changes in crystalline materials, and it is well-suited for studying the changes in zinc anodes and the effects of additives on them. X-ray absorption spectroscopy (XAS) provides structural information about atoms and their surrounding ligands, making it ideal for observing changes in catalyst structure, valence state, and coordination, which are crucial for inferring reaction mechanisms.
Figure 2. (A) X-ray tomography: Gas-filled voids are depicted in blue, while zinc is represented in red[12]; (B) Evolution of zinc particles (red) into pores (green) as time progresses[13]; (C) Dynamics of dendrite growth, dissolution, and regeneration in ZABs employing porous separators[14]. ZABs: Zinc-air batteries.
Figure 3. (A) Raman shift diagram; (B) CO32- peak intensity at different detection points; (C) Intensity ratio of graphite D and G (ID/IG)[15].
Despite the remarkable benefits of low cost, high safety, and superior energy density, the practical implementation of ZABs encounters obstacles, including underperforming cathode catalysts, irreversibility of zinc anode cycling, and electrolyte degradation. Furthermore, the absence of comprehensive theoretical models hinders a complete understanding of the failure mechanisms across diverse ZAB systems. To delve deeper into the reaction processes, in situ and operando characterization techniques play a vital role in capturing material characteristics at different time intervals and potentials. However, the existing in situ/operando devices encounter challenges in achieving an optimal balance between reaction conditions and high-quality detection signals. Consequently, ZAB research should prioritize the chemical stability of battery materials in strongly alkaline environments or electrolytes containing organic additives to prevent interference with battery operation and detection. Key considerations include defining the research scope, amplifying the strength of detected signals for prompt reception, and designing device structures that closely emulate actual battery operating conditions.
Combining multiple characterization techniques allows for the acquisition of complementary information, and integrating temporal and spatial resolution methods with theoretical calculations can provide a comprehensive understanding of ZABs. However, the challenge in utilizing multiple techniques lies in the difficulty of conducting simultaneous testing, as different testing times may lead to inconsistent battery states, thereby affecting the accurate correlation of results. To truly advance the future design and application of ZABs, greater emphasis must be placed on research conducted under in-situ or operando conditions.
This article presents a detailed analysis of in-situ and operando characterization techniques, highlighting their advantages and innovative solutions for ZABs. Future research can further explore new materials and methods to optimize the performance of ZABs, particularly by using advanced characterization techniques to understand their working mechanisms deeply. Enhancing the stability and sustainability of these batteries is also an important research direction. These advancements will help promote the broader application of ZABs in practical scenarios.
DECLARATIONS
Acknowledgments
We sincerely thank all leading chemists and co-workers involved in developing zinc-air batteries.
Authors’ contributions
Wrote the draft manuscript: Chen YX, Chen R
Revised and rewrote some parts of the manuscript: Lu CZ
Availability of data and materials
Not applicable.
Financial support and sponsorship
The authors are thankful for the financial support of the Natural Science Foundation of Fujian Province (2023H0046), the XMIREM autonomously deployment project (2023CX10, 2023GG01), the National Natural Science Foundation of China (22275185), the Major Research Project of Xiamen (3502Z20191015), and the Fujian Science & Technology Innovation Laboratory for Optoelectronic Information of China (2021ZR132, 2021ZZ115).
Conflicts of interest
Chen YX served as a Junior Editorial Member of Chemical Synthesis; he was not involved in the editorial process of the work. While the other authors have declared that they have no conflicts of interest.
Ethical approval and consent to participate
Not applicable.
Consent for publication
Not applicable.
Copyright
© The Author(s) 2024.
REFERENCES
1. Das TK, Jesionek M, Çelik Y, Poater A. Catalytic polymer nanocomposites for environmental remediation of wastewater. Sci Total Environ 2023;901:165772.
2. Das TK, Das NC. Advances on catalytic reduction of 4-nitrophenol by nanostructured materials as benchmark reaction. Int Nano Lett 2022;12:223-42.
3. Poudel MB, Vijayapradeep S, Sekar K, Kim JS, Yoo DJ. Pyridinic-N exclusively enriched CNT-encapsulated NiFe interfacial alloy nanoparticles on knitted carbon fiber cloth as bifunctional oxygen catalysts for biaxially flexible zinc–air batteries. J Mater Chem A 2024;12:10185-95.
4. Karthikeyan S, Sidra S, Ramakrishnan S, et al. Heterostructured NiO/IrO2 synergistic pair as durable trifunctional electrocatalysts towards water splitting and rechargeable zinc-air batteries: an experimental and theoretical study. Appl Catal B Environ 2024;355:124196.
5. Kumar RS, Mannu P, Prabhakaran S, et al. Trimetallic oxide electrocatalyst for enhanced redox activity in zinc-air batteries evaluated by in situ analysis. Adv Sci 2023;10:e2303525.
6. Poudel MB, Balanay MP, Lohani PC, Sekar K, Yoo DJ. Atomic engineering of 3D self-supported bifunctional oxygen electrodes for rechargeable zinc-air batteries and fuel cell applications. Adv Energy Mater 2024;14:2400347.
8. Zhang J, Zhao Z, Xia Z, Dai L. A metal-free bifunctional electrocatalyst for oxygen reduction and oxygen evolution reactions. Nat Nanotechnol 2015;10:444-52.
9. Li L, Tsang YCA, Xiao D, Zhu G, Zhi C, Chen Q. Phase-transition tailored nanoporous zinc metal electrodes for rechargeable alkaline zinc-nickel oxide hydroxide and zinc-air batteries. Nat Commun 2022;13:2870.
10. Chen P, Zhang K, Tang D, et al. Recent progress in electrolytes for Zn-air batteries. Front Chem 2020;8:372.
11. Hosseini S, Masoudi Soltani S, Li Y. Current status and technical challenges of electrolytes in zinc–air batteries: an in-depth review. Chem Eng J 2021;408:127241.
12. Franke-Lang R, Arlt T, Manke I, Kowal J. X-ray tomography as a powerful method for zinc-air battery research. J Power Sources 2017;370:45-51.
13. Hack J, Patel D, Bailey JJ, Iacoviello F, Shearing PR, Brett DJL. In situ x-ray computed tomography of zinc–air primary cells during discharge: correlating discharge rate to anode morphology. J Phys Mater 2022;5:014001.
14. Yufit V, Tariq F, Eastwood DS, et al. Operando visualization and multi-scale tomography studies of dendrite formation and dissolution in zinc batteries. Joule 2019;3:485-502.
Cite This Article
How to Cite
Chen, Y. X.; Chen, R.; Lu, C. Z. Advanced in-situ/operando characterization techniques: aiding the development of zinc-air batteries. Chem. Synth. 2024, 4, 74. http://dx.doi.org/10.20517/cs.2024.109
Download Citation
Export Citation File:
Type of Import
Tips on Downloading Citation
Citation Manager File Format
Type of Import
Direct Import: When the Direct Import option is selected (the default state), a dialogue box will give you the option to Save or Open the downloaded citation data. Choosing Open will either launch your citation manager or give you a choice of applications with which to use the metadata. The Save option saves the file locally for later use.
Indirect Import: When the Indirect Import option is selected, the metadata is displayed and may be copied and pasted as needed.
About This Article
Copyright
Author Biographies



Data & Comments
Data
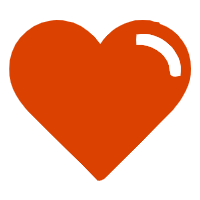
Comments
Comments must be written in English. Spam, offensive content, impersonation, and private information will not be permitted. If any comment is reported and identified as inappropriate content by OAE staff, the comment will be removed without notice. If you have any queries or need any help, please contact us at support@oaepublish.com.