The anticancer activity of quercetin, luteolin, myricetin, and kaempferol in the development of hepatocellular carcinoma: a narrative review
Abstract
Hepatocellular carcinoma (HCC) ranks as the third leading cause of cancer-related deaths worldwide. It presents a significant and rising global health challenge due to its complex development, high mortality rate, and poor prognosis. The primary treatment methods include chemotherapy, radiotherapy, and surgery, yet each is associated with severe side effects. While combination therapies can enhance outcomes, they also tend to amplify the adverse effects. For this reason, the prevention of HCC has a major impact on world health. Current knowledge indicates that a healthy lifestyle combined with a healthy diet is an excellent preventive strategy. In this respect, the Mediterranean diet represents a valid preventive strategy widely recognized by the scientific community. Its effectiveness is proven by the presence of several polyphenols in its main foods, including quercetin, luteolin, myricetin, and kaempferol. These polyphenols are present in different functional foods and show anticancer activity. This narrative review aims to assess the anti-HCC activity of these polyphenols that characterize the Mediterranean diet.
Keywords
INTRODUCTION
Hepatocellular carcinoma (HCC) is the predominant form of primary liver cancer, responsible for about 75%-85% of all liver cancer cases globally[1]. It frequently develops in individuals with chronic liver conditions and cirrhosis, often linked to long-term hepatitis B (HBV) or hepatitis C (HCV) virus infections, excessive alcohol consumption, or non-alcoholic fatty liver disease (NAFLD)[2]. However, it has been reported that 20% of HCC cases can develop in a non-cirrhotic liver[3]. The incidence of HCC has been rising globally, with significant geographic variations. Regions with a high risk of HCC include East Asia, sub-Saharan Africa, and certain parts of Europe, primarily because of the widespread presence of HBV and HCV infections[4]. Key risk factors for HCC include chronic viral hepatitis, heavy alcohol use, and exposure to aflatoxins, metabolic disorders, genetic predispositions, and gut microbiota dysbiosis related to a high-fat diet[5-7]. Preventive measures focus on vaccination against HBV, antiviral treatments, lifestyle modifications to reduce alcohol intake and dysmetabolic comorbidities, as well as screening programs for high-risk populations[8-11]. A resolutory therapeutic approach, besides radiotherapy and chemotherapy, is liver transplantation, a procedure that unfortunately entails long waiting lists. Moreover, current therapies in use can cause serious side effects in patients, especially when combination therapy must be administered to achieve the desired effects[12]. Therefore, applying appropriate prevention strategies is essential and some level of prevention can be achieved with a healthy diet. It is now known that diet plays a crucial role in many diseases concerning the gastrointestinal tract. Indeed, a high-calorie and unbalanced diet, combined with an unregulated lifestyle, are central pathogenetic factors related to the development of NAFLD, with possible subsequent progression into HCC[13-16]. At the same time, much scientific research has shown that a healthy diet protects against several types of cancer[13]. The current state of the art focuses on identifying the effects of individual nutrients on cancer, rather than on whole food regimens[17]. Epidemiological studies indicate that a diet rich in fruits and vegetables may play a protective role against gastrointestinal cancers[13,14]. Notably, consuming more than 100 grams of vegetables per day or including 3-4 servings of vegetables weekly was associated with a marked reduction in HCC risk[18]. Certain types of vegetables, such as celery (P = 0.03), mushrooms (P = 0.03), allium vegetables (e.g., onions, garlic, garlic sprouts) (P < 0.01), legumes and legume-based foods (P = 0.04), squash, and carrots, were found to significantly lower the risk of HCC[19]. In this perspective, the Mediterranean diet is a useful strategy for preventing the occurrence of HCC, as components of the Mediterranean diet, such as antioxidants and anti-inflammatory compounds, contribute to lowering oxidative stress and inflammation, both key drivers of carcinogenesis in the liver. Additionally, the diet’s emphasis on healthy fats and fiber may improve metabolic health, reducing the risk factors for HCC, such as obesity, insulin resistance, and NAFLD. The Mediterranean diet’s overall impact on maintaining liver function, modulating the gut microbiota, and reducing pro-carcinogenic signaling pathways further supports its protective role against HCC development[15,20-22]. This dietary regimen, inspired by the traditional dietary patterns of countries in Southern Italy, gained scientific attention through the pioneering study of Ancel Keys[23]. However, some clarifications on this study must be made: namely, the fact that smoking consumption was underestimated, while daily alcohol consumption was about 84 g/day, and that only high consumption of olive oil was assumed, while in Montegiorgio and Crevalcore (Central-North Italy), in the post-war, the use of animal lard was more frequent[24,25]. This dietary approach emphasizes the intake of fruits, vegetables, whole grains, legumes, nuts, and extra-virgin olive oil, along with moderate consumption of fish, poultry, dairy and limited consumption of red meat and sweets. The health benefits of the Mediterranean diet are attributed to its rich content of polyphenols, fibers, and healthy fats, which collectively contribute to a reduction in inflammation, an improvement of lipid profiles, and an enhancement of cardiovascular health[26]. Polyphenols are a different group of naturally occurring compounds found in plant-based foods, well-known for their antioxidant, anti-inflammatory, and anticancer benefits[27]. Among the numerous polyphenols, quercetin, luteolin, myricetin, and kaempferol stand out due to their potent biological activities: quercetin, abundant in apples, onions, and capers, exhibits strong anti-inflammatory and anticancer effects, while luteolin, found in celery, parsley, and artichokes, is known for its anti-inflammatory and neuroprotective properties[28,29]. Similarly, myricetin, present in berries, nuts, and red wine, has demonstrated significant antioxidant and anticancer activities, while kaempferol, found in beans and tea, is known for its cardioprotective and anticancer effects[30,31]. These polyphenols contribute to the health benefits associated with the Mediterranean diet, offering protective effects against various chronic diseases, including cancer[32,33]. Therefore, this narrative review aims to assess the anti-HCC activity of these polyphenols that characterize the Mediterranean diet.
MATERIALS AND METHODS
We conducted a search on the main database such as PubMed and Medline for original research articles, reviews, meta-analyses, and editorials using a combination of the following keywords, their acronyms, and related terms: hepatocellular carcinoma, HCC, Mediterranean diet, polyphenols, quercetin, luteolin, myricetin, kaempferol, diet, fruits, vegetables, olive oil. The last search was dated 31 May 2024. In this narrative review, studies performed on preclinical models were included to investigate the anti-carcinogenic properties of the four most common polyphenols (quercetin, luteolin, myricetin, and kaempferol) found in foods of the Mediterranean diet, considering each stage of HCC development. Studies conducted on other forms of liver cancer were excluded from our analysis.
QUERCETIN
Quercetin, or 3,5,7,3’,4’-pentahydroxyflavone, is a flavonoid that can be found in high amounts in apples, onions, and capers. Quercetin's chemical structure consists of three benzene rings and five hydroxyl groups. It appears as a yellow crystalline substance, insoluble in cold water but soluble in alcohol and lipids, with a bitter taste. Free radicals react rapidly with other molecules to capture electrons and stabilize themselves, causing the loss of electrons from the other molecule and initiating a chain reaction that can harm living cells. Quercetin’s antioxidant properties are largely due to its hydroxyl groups located at positions 3, 5, 7, 3’, and 4’ on the A and B rings, the double bond between the second and third carbons, and the carbonyl group on the fourth carbon[28]. Furthermore, this flavonoid showed anti-HCC effects in several preclinical models. Specifically, murine models with N-nitroso diethylamine (NDEA)-induced HCC were compared to mice administered NDEA+quercetin and an untreated control group[34]. The results showed a significant decrease in oxidative stress and improvements in liver histology in the group treated with quercetin. This can be attributed to quercetin’s inhibition of lipid peroxidation, leading to the release of glutathione peroxidase, which exerted antioxidant effects and reduced damage. Additionally, quercetin reduced the number of pre-neoplastic lesions in male rats by significantly modulating the expression of the epidermal growth factor receptor compared to the control group. Quercetin has been shown to elevate levels of insulin-like growth factor 1 (IGF-1), which are typically low in HCC patients and associated with poor prognosis[35]. One key mechanism behind quercetin’s anticancer activity involves the NF-E2-related factor 2 (Nrf2) signaling pathway. While transient activation of Nrf2 helps protect liver cells from oxidative stress and potential tumor formation, prolonged activation has the opposite effect[36]. In vivo studies have demonstrated that quercetin significantly reduces HCC growth, angiogenesis, and metastasis in mouse models by targeting persistent Nrf2 activation and regulating the nuclear factor kappa-light-chain-enhancer of activated B cells (NF-κB) signaling[37,38]. A study exploring quercetin’s effect on the NF-κB pathway in HCC cells, in combination with ZD55-tumor necrosis factor-related apoptosis-inducing ligand (TRAIL), a protein that induces tumor cell apoptosis, found that treatment with ZD55-TRAIL and quercetin significantly decreased the expression of IκBα, p65, and p50 proteins, thereby inhibiting NF-κB signaling and promoting apoptosis[39]. Additionally, quercetin triggers autophagy by suppressing the AKT/ mammalian target of rapamycin (mTOR) pathway while activating the mitogen-activated protein kinase (MAPK) pathways: the first is involved in negatively regulating autophagy mechanisms, while the MAPK pathways regulate this process positively[40]. However, this mechanism might partially involve the downregulation of Janus kinase/signal transducer and activator of transcription (JAK2/STAT3), which is known to be involved in liver tumors by promoting the migration and proliferation of cancer cells, as well as angiogenesis and immunosuppression[41]. Usually, among the numerous activities of quercetin, its antioxidant activity is primarily considered. A recent study confirmed this evidence in BALB/c mice with HCC induced by diethylnitrosamine (DEN): quercetin effectively eliminates free radicals by increasing the activity of antioxidant enzymes and the level of glutathione, while also reducing the activity of liver enzymes[42]. Furthermore, the suppression activity of liver cancer cells was confirmed in vitro on thirteen different cell lines, which exhibited cell cycle arrest at various stages of the cell cycle[43]. Finally, the use of quercetin in combination therapies has been evaluated. Regarding a potential therapeutic approach with sorafenib+quercetin, their combined use significantly improved liver histology, liver enzyme levels, and reduced levels of molecules involved in inflammatory and proliferative pathways [such as tumor necrosis factor-alfa (TNF-α), NF-κB, and p53][44]. Similarly, their combined use with immune checkpoint inhibitors (ICI) reduced gut dysbiosis and the tumor microenvironment[45]. It is known that gut dysbiosis can affect the response to ICI treatment[46]. Figure 1 summarizes the different pathways involved in the anti-HCC activity of quercetin.
Figure 1. Summary of the different pathways involved in the anti-HCC activity of quercetin (created with BioRender.com). ↑: increase; ↓: decrease. HCC: Hepatocellular carcinoma; IGF-1: insulin-like growth factor 1; TNF-α: tumor necrosis factor-alfa; IL: interleukin; NF-κB: nuclear factor kappa-light-chain-enhancer of activated B cells; JAK/STAT3: Janus kinase/signal transducer and activator of transcription; IκBα: inhibitor of kappa B alpha; ROS: reactive oxygen species.
LUTEOLIN
Luteolin, or 3’,4’,5,7-tetrahydroxyflavone, is a molecule that has hydroxy groups positioned at 3’, 4’, 5, and 7, found in celery, parsley, and artichokes and is recognized for its neuroprotective and anti-inflammatory effects[29]. However, its anti-HCC properties have also been evaluated in several preclinical studies. Specifically, C57BL/6 mice with DEN-induced HCC were fed an alcoholic diet, a control diet, and one supplemented with 30 mg of luteolin/kg for 21 days. The luteolin-based diet significantly reduced the number of pre-neoplastic lesions and the incidence of inflammatory foci. This phenomenon is likely due to a decreased expression of hepatic sirtuin 1 (SIRT1) protein, which regulates many cellular processes through deacetylation of the transcription factor FoxO1. Increased FoxO1 activity promotes transcription of pro-inflammatory cytokines such as TNF-α and IL-6[47]. However, its anticancer effect may be linked to multiple signaling pathways. A study on various HCC cell lines highlighted how it can trigger apoptotic processes by inducing G1 cell cycle arrest, significantly reducing levels of the cell cycle regulatory factors cyclin E and cyclin-dependent kinase 2 (Cdk2)[48]. Another study performed on SMMC-7721 HCC cell lines confirms this evidence. In particular, it reported a significant increase in caspase 8 and a decrease in B-cell lymphoma 2 (Bcl-2), leading to cell cycle arrest in the G0/G1 phase. As further proof, treatment with chloroquine, which inhibits autophagy, significantly reduced this phenomenon[49]. Many of the findings reported so far are evident in the study by Elgendy et al., where rats with DEN-induced HCC treated with luteolin showed a significant increase in liver enzymes and a reduction in serum albumin levels. At the same time, there was an evident increase in levels of Bcl-2, NF-κB, and transforming growth factor-beta (TGF-β), but a significant decrease in Caspase 3 levels[50]. Moreover, although p53 is extensively involved in tumorigenesis, the effectiveness of luteolin as a potential anti-HCC approach is independent of its action[51]. Finally, luteolin associated with other drugs can drive apoptosis in cancer cells through JNK mediation. The outcome of JNK signaling, whether it promotes cell survival or cell death, depends on the strength and duration of the damage signal[52,53]. Figure 2 summarizes the different pathways involved in the anti-HCC activity of luteolin.
Figure 2. Summary of the different pathways involved in the anti-HCC activity of luteolin (created with BioRender.com). ↑: increase; ↓: decrease. HCC: Hepatocellular carcinoma; SIRT1: sirtuin 1 protein; Cdk2: cyclin-dependent kinase 2; NF-κB: nuclear factor kappa-light-chain-enhancer of activated B cells; TGF: transforming growth factor.
MYRICETIN
Myricetin [3,5,7-trihydroxy-2-(3,4,5-trihydroxyphenyl)-4-chromenone] is one of the most common flavonoids that abound in fruits. Myricetin is abundant in vegetables (such as onions), fruits (especially berries, grapes, and nuts), and tea. Since its discovery in 1896, myricetin has been known for its innumerable biological activities[54,55], some of them being its antithrombotic, antioxidant, anticancer, and anti-inflammatory effects, including blood glucose reduction and liver and kidney protection[56-60]. Furthermore, myricetin exhibits strong anticancer activity against several types of cancer, such as ovarian, gastric, and breast[61-64]. Not all the mechanisms that determine antitumor effects have been clarified. A study by Li et al. on two different cell lines (HepG2 and Huh-7) treated for 72 h with myricetin reported several pieces of evidence on the anticancer effect of myricetin[65]. By administering a dose of 100 and 200 μM of myricetin, a drastic reduction in cell growth and a clear induction of cell death was reported. In addition, cell growth was much slower after 2-3 days of treatment, indicating its anti-HCC effects. Apoptosis levels were also significantly increased following myricetin treatment in both cell lines. In particular, high caspase 3 levels were found in HCC cells treated with myricetin, confirming the reduction in cancer cell proliferation. The authors next sought to identify the molecular mechanism underlying the anticancer activity of myricetin by investigating the gene expression of yes-associated protein/ transcriptional coactivator with PDZ-binding motif (YAP/TAZ) and the kinase activity of large tumor suppressor (LATS)1/2. The YAP/TAZ gene is involved in tumorigenesis and cancer propagation but is degraded through the kinase activity of LATS1/2. The study conducted by Li showed that myricetin promotes the degradation of YAP/TAZ through the regulation of LATS1/2 in both cell lines. A second study was carried out by Yang et al. on two tumor cell types of human hepatocytes Hep3B and HepG2, reporting IC50s of myricetin of 48.473 and 28.147 μM, respectively[66]. The results reported in this study indicate that myricetin facilitates autophagy activation of HCC cells and inhibits HCC growth. Myricetin reduced membrane-associated RING-CH-1 (MARCH 1) concentrations in HCC cells. However, myricetin inhibited MARCH 1 mRNA expression in HepG2 cells but increased it in Hep3B cells. This discrepancy could be attributed to cell specificity. Myricetin, therefore, activates autophagy in HCC cells due to the downregulation of MARCH 1. MARCH 1 could positively regulate the signal transducer and activator of the transcription factor 3 (Stat3) signaling pathway. This investigation demonstrated that myricetin can reduce the expression of p-Stat3, and the inhibition of p-Stat3 may trigger autophagy activation. Additionally, Yang et al. found that myricetin halted the HCC cell cycle at the G2/M phase, thereby inhibiting HCC cell proliferation through autophagy regulation. The authors concluded that myricetin achieves this by upregulating the MARCH 1/p38 MAPK/Stat3 signaling pathway, leading to cell cycle arrest in the G2/M phase. The epithelial-mesenchymal transition (EMT) is important in cancer cell invasion and proliferation[67]. The study conducted by Ma et al. reported the effects of myricetin precisely on the EMT, evaluating cell viability and focusing on changes in cancer cell migration and invasion[68]. Human HCC MHCC97H cell lines were treated with myricetin at concentrations of 25, 50, and 100 μM. According to this study, myricetin inhibited both cell migration and cell invasive capacity after treatment with 100 μM of the compound for 24 and 48 h. In addition, the Authors reported the regulatory activity of myricetin on mRNA expression of genes associated with migration and invasion. Specifically, myricetin treatment seems to inhibit migration and invasion of MHCC97H cells through upregulation of E-cadherin and downregulation of N-cadherin and vimentin. Finally, the study reported that myricetin reduced the number of fibers and weakened filopodia and lamellipodia in MHCC97H cells. These effects on cytoskeleton rearrangement could contribute to the effects of myricetin on cell migration and invasion. The study conducted by Ji et al. reported the pro-apoptotic effects of myricetin on two human HCC cell lines (SMMC-7721 and Hep3B)[69]. The authors underlined that myricetin significantly suppressed cell viability in a dose- and time-dependent manner, demonstrating relative specificity for cancer cells. In the SMMC-7721 and Hep3B HCC cell lines, myricetin induced apoptosis by activating the caspase 9, caspase 3, and Poly (ADP-ribose) polymerase (PARP) signaling cascades. This indicates that myricetin inhibits cancer cells by promoting apoptosis and cell cycle arrest. Additionally, Ji et al. provided initial evidence that myricetin-induced apoptosis in HCC cells is at least partially mediated by ER stress and the downstream C/EBP homologous protein (CHOP) signaling pathway[69]. The study also found that myricetin treatment can induce autophagy by influencing ER stress and unfolded protein response (UPR) signaling pathways, such as serine/threonine-protein kinase/endoribonuclease inositol-requiring enzyme 1 α-c-Jun N-terminal kinases (IRE1α-JNK) and Ca2+- AMP-activated protein kinase (AMPK), which may act as upstream regulators of the mTOR. Finally, this evidence suggested that myricetin exhibits high selectivity for cancer cells. In a research performed by Seydi et al., ten male Sprague Dawley rats were divided into two groups: group A, consisting of normal rats on a standard diet, and group B, rats with induced HCC[70]. Hepatocytes from both groups were observed after administrations at different doses of myricetin (maximum dose 100 μM) at 6-, 12-, and 24-h intervals. In this study, myricetin was shown to selectively increase apoptosis in HCC cells, while healthy hepatocytes reported no significant effects. Specifically, myricetin reduced cell viability (P < 0.05) in rat HCC hepatocytes. Furthermore, myricetin (12.5, 25 and 50 μM) determined its cytotoxic effect on hepatocytes with HCC through significantly increased levels of ROS, mitochondrial swelling, mitochondrial membrane permeability, and cytochrome c release in mitochondria. Finally, the Authors reported increased caspase 3 activation and apoptosis only in HCC hepatocytes. Figure 3 summarizes the different pathways involved in the anti-HCC activity of myricetin.
Figure 3. Summary of the different pathways involved in the anti-HCC activity of myricetin (created with BioRender.com). ↑: increase; ↓: decrease. HCC: Hepatocellular carcinoma; YAP/TAZ: yes-associated protein/ transcriptional coactivator with PDZ-binding motif; MARCH1: membrane-associated RING-CH-1; PARP: poly (ADP-ribose) polymerases; ROS: reactive oxygen species; ER: endoplasmic reticulum.
KAEMPFEROL
Kaempferol (3,4’,5,7-tetrahydroxyflavone) is a natural flavonoid found in several medicinal herbs and fruits[71]. This polyphenol has been shown to reduce the risk of various diseases, such as cardiovascular disorders, diabetes, and several infectious diseases, and possesses anti-inflammatory, anti-osteoarthritic, and anti-asthmatic activities[72-76]. In addition, kaempferol has been shown to possess several anticancer effects, including preventing metastasis in oral cancer and inducing apoptosis of colorectal, breast and prostate cancer, and leukemia cells[77-81]. Several studies have been conducted to evaluate the effects of kaempferol on HCC. The study by Guo et al. was conducted on human HepG2 liver cancer cell lines and demonstrated the ability of kaempferol to induce apoptosis in a dose- and time-dependent manner[82]. The authors also reported increased transcriptional and protein levels of glucose-regulated protein (GRP) 78, GRP94 and CHOP, factors involved in ER stress. These results suggest that increased ER stress is one of the mechanisms induced by kaempferol to initiate apoptosis, particularly through the CHOP signaling pathway. An additional study by Guo et al. investigated the effects of kaempferol on the autophagy of cancer cells in HCC[83]. The study was conducted on HepG2 and Huh 7 of human liver cancer cells. This study reported a strong increase in Atg5, Atg7, Beclin1 proteins and Microtubule-associated protein light chain 3-I (LC3-I) to LC3-II conversion, all factors related to autophagy, after kaempferol administration. Furthermore, the Authors posited that the CHOP signaling pathway, activated by kaempferol, also plays a key role in regulating autophagy, correlating apoptosis, autophagy, and endoplasmic reticulum (ER) stress with each other. Yang et al. analyzed the effects of kaempferol on several liver cancer cell lines: Huh-7, Huh-1, HepG2, HepG2.2.15, SK-Hep-1, PLC/PRF/5, human hepatoma cell line (HLE), primary lung fibroblast, normal, human (HLF), and Hep3B[84]. The viability of treated cells and their growth were significantly reduced by kaempferol treatment. Comparing the effects of kaempferol, doxorubicin, and their combination against a control group of HepG2 cells, it was observed that the combination of kaempferol and doxorubicin showed significantly stronger growth inhibition than the individual compounds (P < 0.05). Interesting results revealed that kaempferol modifies the protein expression of critical factors involved in signaling pathways that activate mitochondrial apoptosis, resulting in the activation of its pathway. Combined treatment (kaempferol and doxorubicin) also revealed higher inhibitory activity on apoptosis-, migration-, and invasion-related proteins [including Matrix metalloproteinase 2 (MMP-2), MMP-9, Phosphoinositide 3-kinase (PI3K), Akt, mTOR and S6K] than single treatments. The study conducted by Ju et al. also reported the effects of kaempferol on cancer cell migration and invasion[85]. The authors analyzed two human liver tumor cell lines, Huh-7 and SK-Hep-1. Treatment with kaempferol (25 μM) showed a clear reduction in cell migration and invasion capacity in both cell lines. The authors subsequently reported the activity of kaempferol on the expressed protein levels of MMP-9, cathepsin D, and cathepsin C, factors involved in cancer cell migration and invasion capacity. In particular, a significant inhibitory action of kaempferol on MMP-9 activity was observed in Huh-7 and SK-Hep-1 cells. The study reported that the observed reduction in invasion capacity may be due to the reduction in phosphorylation of Akt by kaempferol. These results indicate that kaempferol, by reducing Akt activation, modulates MMP-9 expression and cell invasion, thus exhibiting anti-metastatic activity. The antitumor effects of kaempferol were also reported in the study conducted by Han et al.[86]. This analysis demonstrated the antitumor activity of kaempferol on several human liver cancer cell lines (HepG2, Huh-7, BEL7402 and SMMC) with an IC50 of kaempferol between 25-50 μM. Kaempferol can also inhibit cell proliferation by cell cycle arrest in the G1 phase on HepG2 cells. Furthermore, this study reported that the cell death caused by kaempferol was not related to the apoptotic process but to the initiation of autophagy. The Authors observed that kaempferol treatment significantly activated AMPK. Kaempferol-activated AMPk (50 μM) consequently resulted in the phosphorylation of unc-51-like autophagy activating kinase 1 (Ulk1), upregulation of Beclin-1/Atg-5, and degradation of p62, as well as conversion of LC3B-I to LC3B-II and formation of LC3B dots. A critical factor in the development of HCC is the formation of hypoxic regions, which arise from increased cell proliferation and an inadequate blood supply. Hypoxia can accelerate HCC progression by promoting angiogenesis and metabolic adaptation[87]. A central player in the cellular response to low oxygen levels is hypoxia-inducible factor 1 (HIF-1), a transcription factor that regulates genes involved in survival, proliferation, angiogenesis, invasion, and metastasis[88]. Elevated HIF-1α expression in HCC has been linked to VEGF secretion and malignant transformation[89-91]. Mylonis et al. demonstrated that kaempferol effectively inhibits the viability of hepatoma cancer cells under hypoxic conditions[92]. In their study on human Huh7 liver cancer cells modified to simulate hypoxia, kaempferol significantly suppressed HIF-1 transcriptional activity in a dose-dependent manner. In addition, the same dose of kaempferol was also observed to inhibit the MAPK signaling pathway, which is also involved in cellular adaptation to hypoxia. This study underscores how the inhibitory potential of kaempferol is much more pronounced under hypoxic conditions than in normal cellular oxygen concentrations. Figure 4 summarizes the different pathways involved in the anti-HCC activity of kaempferol.
Figure 4. Summary of the different pathways involved in the anti-HCC activity kaempferol (created with BioRender.com). ↑: increase; ↓: decrease. HCC: Hepatocellular carcinoma; GRP78: glucose-regulated protein 78; GRP94: glucose-regulated protein 94; CHOP: C/EBP homologous protein; LC3: light chain 3; MMP: matrix metalloproteinase; PI3K: phosphoinositide 3-kinase; mTOR: mammalian target of rapamycin; S6K: S6 kinase; AMPK: AMP-activated protein kinase; HIF-1: hypoxia-inducible factor 1; MAPK: mitogen-activated protein kinase; ER: endoplasmic reticulum.
Overall, the four molecules investigated showed numerous biological effects affecting HCC through the activation of specific molecules and pathways, as summarized in Table 1.
Summary of the different pathways involved in the anti-HCC effects of polyphenols
Pathways | Biological activity | References |
↑E-cadherin ↓JAK2/STAT3, vimentin, MMP-2, MMP-9, PI3K, Akt, mTOR, S6K | Cell invasion and migration | [28,55,71,72] |
↑IGF-1, NF-κB, caspase 8, PARP, GRP78, GRP94, CHOP ↓IκBα, p65, p50, Bcl-2 | Apoptosis | [22,26,36,55,69] |
↓JAK2/STAT3, MARCH 1, cyclin E, Cdk2, YAP/TAZ | Cell proliferation | [28,35,52,53] |
↑TNF-α, IL-6, IL-17A, NF-κB ↓SIRT1 | Inflammation | [25,34,36] |
DISCUSSION
The present narrative review has explored the anti-HCC potential of specific polyphenols, namely quercetin, luteolin, myricetin, and kaempferol, that are found abundantly in the foods of the Mediterranean diet. Indeed, diet represents a crucial junction in the prevention of HCC. Recent studies report that a high-calorie, unbalanced diet can increase the risk of cancer occurrence; in contrast, a healthy, balanced diet reduces its incidence[6]. The current composition of the Mediterranean diet is an attractive prevention strategy due to its relatively high intake of vegetables and fruits[27]. These foods are particularly rich in polyphenols, the most prevalent being precisely quercetin, luteolin, myricetin and kaempferol. This work differentiates itself from other recently published studies by providing a comprehensive synthesis that uniquely focuses on the molecular mechanisms behind the anti-HCC effects of these polyphenols. While previous studies have often examined the general health benefits of the Mediterranean diet or the effects of individual polyphenols on various cancers, this review specifically targets their role in HCC, a type of cancer with increasing global prevalence and limited therapeutic options. The present review of literature offers detailed insights into the molecular pathways through which polyphenols exert their anti-HCC effects. By integrating data from multiple studies, it identifies common and distinct pathways modulated by these polyphenols, such as the suppression of NF-κB signaling, the initiation of autophagy, and the promotion of apoptosis. This synthesis of information provides a clearer understanding of how these compounds could be utilized in potential therapeutic strategies of HCC. Unlike many studies that focus on isolated compounds, this review highlights the potential synergistic effects of combining polyphenols with conventional therapies like ICI. It emphasizes the possibility of enhancing therapeutic efficacy while reducing adverse effects through such combinations. From this perspective, polyphenols assume an important role in the prevention of HCC since they could act in multiple cellular stages and different mechanisms, suggesting a potential synergistic antitumor action. Scientific research is paying much attention to polyphenols and their effects, some of which are also related to aging, age-related diseases, and oxidative stress[93]. Despite the promising findings, several criticisms and challenges exist within this field of study. Firstly, much of the evidence is derived from preclinical models, including cell lines and animal studies. The translation of these findings into clinical practice remains uncertain, as human studies are limited and often fail to replicate the efficacy observed in preclinical settings. This highlights the need for well-designed clinical trials to confirm the therapeutic potential of these polyphenols in humans. Secondly, another criticism lies in the potential variability in polyphenol content found in different food sources, which can be influenced by different factors such as agricultural practices, food processing, and preparation methods. This variability complicates the standardization of polyphenol intake in both research and dietary recommendations. At the same time, it is important to remember that the positive effects of the mentioned polyphenols from consuming fruits, vegetables, and olive oil (100 calories/spoon) may be limited if people continue to follow a high-calorie diet that includes unclear amounts of alcoholic beverages, which are major contributors to liver damage, impaired insulin sensitivity, elevated blood glucose levels, and increased insulin concentrations[94,95].
CONCLUSIONS
In conclusion, the growing evidence highlights the role of polyphenols, particularly quercetin, luteolin, myricetin, and kaempferol, in the prevention and treatment of HCC. These bioactive compounds, commonly found in Mediterranean food and its related diet, demonstrate multifaceted anticancer properties. Through mechanisms such as antioxidant activity, the modulation of cellular signaling pathways (e.g., NF-κB, AKT/mTOR, JAK2/STAT3), and the modulation of apoptosis and autophagy, these polyphenols contribute to a reduction in tumorigenesis, cancer cell proliferation, and metastasis in preclinical models. Additionally, they show promising results when used in combination with conventional therapies, such as ICI, enhancing therapeutic outcomes and minimizing adverse effects. The Mediterranean diet’s protective role against HCC may, therefore, be attributed to the synergistic effects of these polyphenols, which work collectively to reduce inflammation, oxidative stress, and promote healthy liver function. Despite these encouraging findings, additional clinical trials are necessary to confirm the therapeutic effectiveness and safety of these compounds in human subjects. Establishing optimal dosages, long-term benefits, and potential side effects is essential for translating these discoveries into clinical practice. Nevertheless, these findings support the implementation of dietary strategies, such as increased adherence to the Mediterranean diet, as a preventive measure against HCC development, particularly among populations at high risk.
DECLARATIONS
Acknowledgments
We would like to thank Simone Scarlata for his critical review of the English language.
Authors’ contributions
Wrote, reviewed, and edited the manuscript: Paravati MR, Scarlata GGM
Reviewed and approved the final manuscript as submitted: Milanović M, Milić N
Conceptualized and designed the review: Abenavoli L
Availability of data and materials
Not applicable.
Financial support and sponsorship
None.
Conflicts of interest
All authors declared that there are no conflicts of interest.
Ethical approval and consent to participate
Not applicable.
Consent for publication
Not applicable.
Copyright
© The Author(s) 2024.
REFERENCES
1. Sayiner M, Golabi P, Younossi ZM. Disease burden of hepatocellular carcinoma: a global perspective. Dig Dis Sci 2019;64:910-7.
2. Reddy KR, McLerran D, Marsh T, et al. Incidence and risk factors for hepatocellular carcinoma in cirrhosis: the multicenter hepatocellular carcinoma early detection strategy (HEDS) study. Gastroenterology 2023;165:1053-1063.e6.
3. Desai A, Sandhu S, Lai JP, Sandhu DS. Hepatocellular carcinoma in non-cirrhotic liver: a comprehensive review. World J Hepatol 2019;11:1-18.
4. Samant H, Amiri HS, Zibari GB. Addressing the worldwide hepatocellular carcinoma: epidemiology, prevention and management. J Gastrointest Oncol 2021;12:S361-73.
5. Ge XY, Sun MC, Wang TY, et al. Analysis of risk factors of hepatocellular carcinoma and establishment of a clinical prognosis model. Front Oncol 2023;13:1067353.
6. Scarlata GGM, Cicino C, Spagnuolo R, et al. Impact of diet and gut microbiota changes in the development of hepatocellular carcinoma. Hepatoma Res 2024;10:19.
7. Colaci C, Gambardella ML, Maria Scarlata GG, et al. Dysmetabolic comorbidities and non-alcoholic fatty liver disease: a stairway to metabolic dysfunction-associated steatotic liver disease. Hepatoma Res 2024;10:16.
8. Marascio N, Pavia G, Romeo I, et al. Real-life 3D therapy failure: analysis of NS5A 93H RAS plus 108 K polymorphism in complex with ombitasvir by molecular modeling. J Med Virol 2018;90:1257-63.
9. Romeo I, Marascio N, Pavia G, et al. Structural modeling of new polymorphism clusters of HCV polymerase isolated from direct - acting antiviral naïve patients: focus on dasabuvir and setrobuvir binding affinity. ChemistrySelect 2018;3:6009-17.
10. Marascio N, Mazzitelli M, Pavia G, et al. Clinical, virological characteristics, and outcomes of treatment with sofosbuvir/ledipasvir in two pediatric patients infected by HCV genotype 4. Cells 2019;8:416.
11. El-Serag HB, Ward JW, Asrani SK, et al. Prevention of hepatocellular carcinoma (HCC). White paper of the texas collaborative center for hepatocellular cancer (TeCH) multi-stakeholder conference. Clin Gastroenterol Hepatol 2023;21:2183-92.
12. Abenavoli L, Scarlata GGM, Paravati MR, Boccuto L, Luzza F, Scarpellini E. Gut microbiota and liver transplantation: immune mechanisms behind the rejection. Biomedicines 2023;11:1792.
13. Key TJ, Schatzkin A, Willett WC, Allen NE, Spencer EA, Travis RC. Diet, nutrition and the prevention of cancer. Public Health Nutr 2004;7:187-200.
14. Vogtmann E, Xiang YB, Li HL, et al. Fruit and vegetable intake and the risk of colorectal cancer: results from the Shanghai men’s health study. Cancer Causes Control 2013;24:1935-45.
15. Turati F, Trichopoulos D, Polesel J, et al. Mediterranean diet and hepatocellular carcinoma. J Hepatol 2014;60:606-11.
16. Montella M, Crispo A, Giudice A. HCC, diet and metabolic factors: diet and HCC. Hepat Mon 2011; 11:159-62.
17. George ES, Sood S, Broughton A, et al. The association between diet and hepatocellular carcinoma: a systematic review. Nutrients 2021;13:172.
18. Bamia C, Lagiou P, Jenab M, et al. Fruit and vegetable consumption in relation to hepatocellular carcinoma in a multi-centre, European cohort study. Br J Cancer 2015;112:1273-82.
19. Zhang W, Xiang YB, Li HL, et al. Vegetable-based dietary pattern and liver cancer risk: results from the Shanghai women’s and men’s health studies. Cancer Sci 2013;104:1353-61.
20. Bogumil D, Park SY, Le Marchand L, et al. High-quality diets are associated with reduced risk of hepatocellular carcinoma and chronic liver disease: the multiethnic cohort. Hepatol Commun 2019;3:437-47.
21. Li WQ, Park Y, McGlynn KA, et al. Index-based dietary patterns and risk of incident hepatocellular carcinoma and mortality from chronic liver disease in a prospective study. Hepatology 2014;60:588-97.
22. Ma Y, Yang W, Simon TG, et al. Dietary patterns and risk of hepatocellular carcinoma among U.S. men and women. Hepatology 2019;70:577-86.
23. Keys A, Menotti A, Aravanis C, et al. The seven countries study: 2,289 deaths in 15 years. Prev Med 1984;13:141-54.
24. Farchi G, Fidanza F, Mariotti S, Menotti A. Alcohol and mortality in the Italian rural cohorts of the seven countries study. Int J Epidemiol 1992;21:74-81.
25. Farchi G, Fidanza F, Giampaoli S, Mariotti S, Menotti A. Alcohol and survival in the Italian rural cohorts of the seven countries study. Int J Epidemiol 2000;29:667-71.
26. Schwingshackl L, Morze J, Hoffmann G. Mediterranean diet and health status: active ingredients and pharmacological mechanisms. Br J Pharmacol 2020;177:1241-57.
27. Abenavoli L, Procopio AC, Paravati MR, et al. Mediterranean diet: the beneficial effects of lycopene in non-alcoholic fatty liver disease. J Clin Med 2022;11:3477.
28. Aghababaei F, Hadidi M. Recent advances in potential health benefits of quercetin. Pharmaceuticals (Basel) 2023;16:1020.
29. Wang Z, Zeng M, Wang Z, Qin F, Chen J, He Z. Dietary luteolin: a narrative review focusing on its pharmacokinetic properties and effects on glycolipid metabolism. J Agric Food Chem 2021;69:1441-54.
30. Imran M, Saeed F, Hussain G, et al. Myricetin: a comprehensive review on its biological potentials. Food Sci Nutr 2021;9:5854-68.
31. Alam W, Khan H, Shah MA, Cauli O, Saso L. Kaempferol as a dietary anti-inflammatory agent: current therapeutic standing. Molecules 2020;25:4073.
32. Paravati MR, Procopio AC, Milanović M, et al. Onion polyphenols as multi-target-directed ligands in MASLD: a preliminary molecular docking study. Nutrients 2024;16:1226.
33. Procopio AC, Paravati MR, Scarlata GGM, et al. Is the mediterranean diet a good preventive measure for NASH? Hepatoma Res 2023;10:9.
34. Seufi AM, Ibrahim SS, Elmaghraby TK, Hafez EE. Preventive effect of the flavonoid, quercetin, on hepatic cancer in rats via oxidant/antioxidant activity: molecular and histological evidences. J Exp Clin Cancer Res 2009;28:80.
35. Carrasco-Torres G, Monroy-Ramírez HC, Martínez-Guerra AA, et al. Quercetin reverses rat liver preneoplastic lesions induced by chemical carcinogenesis. Oxid Med Cell Longev 2017;2017:4674918.
36. Raghunath A, Sundarraj K, Arfuso F, Sethi G, Perumal E. Dysregulation of Nrf2 in hepatocellular carcinoma: role in cancer progression and chemoresistance. Cancers (Basel) 2018;10:481.
37. Guang LH, Jun GH, Feng LF, Liu J. Quercitrin suppresses hepatocellular carcinoma. Available from: https://www.oncotarget.com/article/23777/text/. [Last accessed on 10 Oct 2024].
38. Wu R, Zhou T, Xiong J, et al. Quercetin, the ingredient of xihuang pills, inhibits hepatocellular carcinoma by regulating autophagy and macrophage polarization. Front Biosci (Landmark Ed) 2022;27:323.
39. Zou H, Zheng YF, Ge W, Wang SB, Mou XZ. Synergistic anti-tumour effects of quercetin and oncolytic adenovirus expressing TRAIL in human hepatocellular carcinoma. Sci Rep 2018;8:2182.
40. Ji Y, Li L, Ma YX, et al. Quercetin inhibits growth of hepatocellular carcinoma by apoptosis induction in part via autophagy stimulation in mice. J Nutr Biochem 2019;69:108-19.
41. Wu L, Li J, Liu T, et al. Quercetin shows anti-tumor effect in hepatocellular carcinoma LM3 cells by abrogating JAK2/STAT3 signaling pathway. Cancer Med 2019;8:4806-20.
42. Marbaniang C, Myrthong E, Priya S, et al. Evaluation of antioxidant properties of quercetin in DEN-induced hepatocellular carcinoma in BALB/c mice. The NEHU J 2019;27:94-106. Available from: https://api.semanticscholar.org/CorpusID:220632067. [Last accessed on 10 Oct 2024].
43. Hisaka T, Sakai H, Sato T, et al. Quercetin suppresses proliferation of liver cancer cell lines in vitro. Anticancer Res 2020;40:4695-700.
44. Abdu S, Juaid N, Amin A, Moulay M, Miled N. Effects of sorafenib and quercetin alone or in combination in treating hepatocellular carcinoma: in vitro and in vivo approaches. Molecules 2022;27:8082.
45. Wu R, Xiong J, Zhou T, et al. Quercetin/anti-PD-1 antibody combination therapy regulates the gut microbiota, impacts macrophage immunity and reshapes the hepatocellular carcinoma tumor microenvironment. Front Biosci (Landmark Ed) 2023;28:327.
46. Abenavoli L, Montori M, Svegliati Baroni G, et al. Perspective on the role of gut microbiome in the treatment of hepatocellular carcinoma with immune checkpoint inhibitors. Medicina (Kaunas) 2023;59:1427.
47. Rafacho BP, Stice CP, Liu C, Greenberg AS, Ausman LM, Wang XD. Inhibition of diethylnitrosamine-initiated alcohol-promoted hepatic inflammation and precancerous lesions by flavonoid luteolin is associated with increased sirtuin 1 activity in mice. Hepatobiliary Surg Nutr 2015;4:124-34.
48. Yee SB, Choi HJ, Chung SW, et al. Growth inhibition of luteolin on HepG2 cells is induced via p53 and Fas/Fas-ligand besides the TGF-β pathway. Int J Oncol 2015;47:747-54.
49. Cao Z, Zhang H, Cai X, et al. Luteolin promotes cell apoptosis by inducing autophagy in hepatocellular carcinoma. Cell Physiol Biochem 2017;43:1803-12.
50. Elgendy A, Abo zaid O, Abd elhamid O, Mansour S. The potential therapeutic effect of luteolin against Diethyl nitrosamine induced hepatocellular carcinoma in rats. Benha Vet Med J 2019;36:264-73.
51. Lee Y, Kwon YH. Regulation of apoptosis and autophagy by luteolin in human hepatocellular cancer Hep3B cells. Biochem Biophys Res Commun 2019;517:617-22.
52. Feng XQ, Rong LW, Wang RX, et al. Luteolin and sorafenib combination kills human hepatocellular carcinoma cells through apoptosis potentiation and JNK activation. Oncol Lett 2018;16:648-53.
53. Nazim UM, Park SY. Luteolin sensitizes human liver cancer cells to TRAILinduced apoptosis via autophagy and JNKmediated death receptor 5 upregulation. Int J Oncol 2019;54:665-72.
54. Semwal DK, Semwal RB, Combrinck S, Viljoen A. Myricetin: a dietary molecule with diverse biological activities. Nutrients 2016;8:90.
55. Harnly JM, Doherty RF, Beecher GR, et al. Flavonoid content of U.S. fruits, vegetables, and nuts. J Agric Food Chem 2006;54:9966-77.
56. Tong Y, Zhou XM, Wang SJ, Yang Y, Cao YL. Analgesic activity of myricetin isolated from Myrica rubra Sieb. et Zucc. leaves. Arch Pharm Res 2009;32:527-33.
57. Yuan X, Liu Y, Hua X, et al. Myricetin ameliorates the symptoms of collagen-induced arthritis in mice by inhibiting cathepsin K activity. Immunopharmacol Immunotoxicol 2015;37:513-9.
58. Kandasamy N, Ashokkumar N. Protective effect of bioflavonoid myricetin enhances carbohydrate metabolic enzymes and insulin signaling molecules in streptozotocin-cadmium induced diabetic nephrotoxic rats. Toxicol Appl Pharmacol 2014;279:173-85.
59. Jose J, Dhanya AT, Haridas KR, et al. Structural characterization of a novel derivative of myricetin from Mimosa pudica as an anti-proliferative agent for the treatment of cancer. Biomed Pharmacother 2016;84:1067-77.
60. Feng J, Chen X, Wang Y, et al. Myricetin inhibits proliferation and induces apoptosis and cell cycle arrest in gastric cancer cells. Mol Cell Biochem 2015;408:163-70.
61. Zheng AW, Chen YQ, Zhao LQ, Feng JG. Myricetin induces apoptosis and enhances chemosensitivity in ovarian cancer cells. Oncol Lett 2017;13:4974-8.
62. Jiao D, Zhang XD. Myricetin suppresses p21-activated kinase 1 in human breast cancer MCF-7 cells through downstream signaling of the β-catenin pathway. Oncol Rep 2016;36:342-8.
63. Jung SK, Lee KW, Byun S, et al. Myricetin suppresses UVB-induced skin cancer by targeting Fyn. Cancer Res 2008;68:6021-9.
64. Yang C, Lim W, Bazer FW, Song G. Myricetin suppresses invasion and promotes cell death in human placental choriocarcinoma cells through induction of oxidative stress. Cancer Lett 2017;399:10-9.
65. Li M, Chen J, Yu X, et al. Myricetin suppresses the propagation of hepatocellular carcinoma via down-regulating expression of YAP. Cells 2019;8:358.
66. Yang W, Su J, Li M, et al. Myricetin induces autophagy and cell cycle arrest of HCC by inhibiting MARCH1-regulated Stat3 and p38 MAPK signaling pathways. Front Pharmacol 2021;12:709526.
67. Ricciardi M, Zanotto M, Malpeli G, et al. Epithelial-to-mesenchymal transition (EMT) induced by inflammatory priming elicits mesenchymal stromal cell-like immune-modulatory properties in cancer cells. Br J Cancer 2015;112:1067-75.
68. Ma H, Zhu L, Ren J, et al. Myricetin inhibits migration and invasion of hepatocellular carcinoma MHCC97H cell line by inhibiting the EMT process. Oncol Lett 2019;18:6614-20.
69. Ji A, Hu L, Ma D, et al. Myricetin induces apoptosis and protective autophagy through endoplasmic reticulum stress in hepatocellular carcinoma. Evid Based Complement Alternat Med 2022;2022:3115312.
70. Seydi E, Rasekh HR, Salimi A, Mohsenifar Z, Pourahmad J. Myricetin selectively induces apoptosis on cancerous hepatocytes by directly targeting their mitochondria. Basic Clin Pharmacol Toxicol 2016;119:249-58.
71. Holland TM, Agarwal P, Wang Y, et al. Dietary flavonols and risk of Alzheimer dementia. Neurology 2020;94:e1749-56.
72. Veeresham C, Rama Rao A, Asres K. Aldose reductase inhibitors of plant origin. Phytother Res 2014;28:317-33.
73. Khalil MI, Sulaiman SA. The potential role of honey and its polyphenols in preventing heart diseases: a review. Afr J Tradit Complement Altern Med 2010;7:315-21.
74. Chien HW, Wang K, Chang YY, et al. Kaempferol suppresses cell migration through the activation of the ERK signaling pathways in ARPE-19 cells. Environ Toxicol 2019;34:312-8.
75. Zhuang Z, Ye G, Huang B. Kaempferol alleviates the interleukin-1β-induced inflammation in rat osteoarthritis chondrocytes via suppression of NF-κB. Med Sci Monit 2017;23:3925-31.
76. Shin D, Park SH, Choi YJ, et al. Dietary compound kaempferol inhibits airway thickening induced by allergic reaction in a bovine serum albumin-induced model of asthma. Int J Mol Sci 2015;16:29980-95.
77. Lin CW, Chen PN, Chen MK, et al. Kaempferol reduces matrix metalloproteinase-2 expression by down-regulating ERK1/2 and the activator protein-1 signaling pathways in oral cancer cells. PLoS One 2013;8:e80883.
78. Li W, Du B, Wang T, Wang S, Zhang J. Kaempferol induces apoptosis in human HCT116 colon cancer cells via the ataxia-telangiectasia mutated-p53 pathway with the involvement of p53 upregulated modulator of apoptosis. Chem Biol Interact 2009;177:121-7.
79. Deepa M, Sureshkumar T, Satheeshkumar PK, Priya S. Antioxidant rich Morus alba leaf extract induces apoptosis in human colon and breast cancer cells by the downregulation of nitric oxide produced by inducible nitric oxide synthase. Nutr Cancer 2013;65:305-10.
80. Szliszka E, Zydowicz G, Janoszka B, Dobosz C, Kowalczyk-Ziomek G, Krol W. Ethanolic extract of Brazilian green propolis sensitizes prostate cancer cells to TRAIL-induced apoptosis. Int J Oncol 2011;38:941-53.
81. Nandi D, Besra SE, Vedasiromoni JR, Giri VS, Rana P, Jaisankar P. Anti-leukemic activity of Wattakaka volubilis leaf extract against human myeloid leukemia cell lines. J Ethnopharmacol 2012;144:466-73.
82. Guo H, Ren F, Zhang L, et al. Kaempferol induces apoptosis in HepG2 cells via activation of the endoplasmic reticulum stress pathway. Mol Med Rep 2016;13:2791-800.
83. Guo H, Lin W, Zhang X, et al. Kaempferol induces hepatocellular carcinoma cell death via endoplasmic reticulum stress-CHOP-autophagy signaling pathway. Oncotarget 2017;8:82207-16.
84. Yang G, Xing J, Aikemu B, Sun J, Zheng M. Kaempferol exhibits a synergistic effect with doxorubicin to inhibit proliferation, migration, and invasion of liver cancer. Oncol Rep 2021;45:32.
85. Ju PC, Ho YC, Chen PN, et al. Kaempferol inhibits the cell migration of human hepatocellular carcinoma cells by suppressing MMP-9 and Akt signaling. Environ Toxicol 2021;36:1981-9.
86. Han B, Yu YQ, Yang QL, Shen CY, Wang XJ. Kaempferol induces autophagic cell death of hepatocellular carcinoma cells via activating AMPK signaling. Oncotarget 2017;8:86227-39.
87. Wu XZ, Xie GR, Chen D. Hypoxia and hepatocellular carcinoma: the therapeutic target for hepatocellular carcinoma. J Gastroenterol Hepatol 2007;22:1178-82.
88. Semenza GL. Defining the role of hypoxia-inducible factor 1 in cancer biology and therapeutics. Oncogene 2010;29:625-34.
89. Huang GW, Yang LY, Lu WQ. Expression of hypoxia-inducible factor 1alpha and vascular endothelial growth factor in hepatocellular carcinoma: impact on neovascularization and survival. World J Gastroenterol 2005;11:1705-8.
90. Tanaka H, Yamamoto M, Hashimoto N, et al. Hypoxia-independent overexpression of hypoxia-inducible factor 1alpha as an early change in mouse hepatocarcinogenesis. Cancer Res 2006;66:11263-70.
91. Yao DF, Jiang H, Yao M, Li YM, Gu WJ et al. Quantitative analysis of hepatic hypoxia-inducible factor-1alpha and its abnormal gene expression during the formation of hepatocellular carcinoma. Hepatobiliary Pancreat Dis Int ;8:407-13.
92. Mylonis I, Lakka A, Tsakalof A, Simos G. The dietary flavonoid kaempferol effectively inhibits HIF-1 activity and hepatoma cancer cell viability under hypoxic conditions. Biochem Biophys Res Commun 2010;398:74-8.
93. Cassotta M, Quiles JL, Giampieri F, Battino M. Aging, age-related diseases, oxidative stress and plant polyphenols: is this a true relationship? MNM 2024;17:255-9.
94. Wang Y, Luo Q, Li Y, et al. Quality assessment of clinical practice guidelines on the treatment of hepatocellular carcinoma or metastatic liver cancer. PLoS One 2014;9:e103939.
Cite This Article
How to Cite
Paravati, M. R.; Scarlata, G. G. M.; Milanović M.; Milić N. ?.; Abenavoli, L. The anticancer activity of quercetin, luteolin, myricetin, and kaempferol in the development of hepatocellular carcinoma: a narrative review. Hepatoma. Res. 2024, 10, 43. http://dx.doi.org/10.20517/2394-5079.2024.86
Download Citation
Export Citation File:
Type of Import
Tips on Downloading Citation
Citation Manager File Format
Type of Import
Direct Import: When the Direct Import option is selected (the default state), a dialogue box will give you the option to Save or Open the downloaded citation data. Choosing Open will either launch your citation manager or give you a choice of applications with which to use the metadata. The Save option saves the file locally for later use.
Indirect Import: When the Indirect Import option is selected, the metadata is displayed and may be copied and pasted as needed.
About This Article
Copyright
Data & Comments
Data
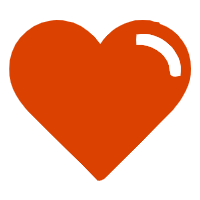
Comments
Comments must be written in English. Spam, offensive content, impersonation, and private information will not be permitted. If any comment is reported and identified as inappropriate content by OAE staff, the comment will be removed without notice. If you have any queries or need any help, please contact us at support@oaepublish.com.