Liquid biopsy-based technologies: a promising tool for biomarker identification in her2-low breast cancer patients for improved therapeutic outcomes
Abstract
The molecular classification of breast cancer plays a pivotal role in developing personalized treatment strategies, with the aim of improving therapeutic outcomes. Despite significant advancements, current diagnostic workflows are hindered by several challenges, including pre-analytical variability, interpretive ambiguity, and inconsistencies in threshold definitions, particularly in cases involving human epidermal growth factor receptor 2 (HER2)-low breast cancer. In this context, liquid biopsy technologies have emerged as promising tools for refining breast cancer diagnostics. Techniques such as circulating tumor DNA and circulating tumor cell analysis provide a non-invasive approach to assessing tumor-associated biomarkers. These methodologies are particularly advantageous for analyzing low-abundance materials, such as formalin-fixed paraffin-embedded samples and liquid biopsies, thus enhancing the precision of molecular classification and informing more targeted therapeutic decisions for breast cancer patients. This review aims to explore the potential of liquid biopsy in addressing the limitations of current diagnostic practices, with a specific focus on its application in HER2-low breast cancer. Furthermore, it advocates for a transition toward high-throughput RNA-based screening and quantification, which may address critical unmet clinical needs.
Keywords
INTRODUCTION
Breast cancer is the most prevalent malignancy among women globally and remains one of the leading causes of cancer-related mortality[1]. The molecular classification of breast cancer plays an essential role in developing personalized treatment strategies, ultimately improving patient outcomes[2]. Historically, breast cancer has been categorized based on the expression of hormone receptors and human epidermal growth factor receptor 2 (HER2), classifications that have been instrumental in guiding the application of targeted therapies[2].
However, recent advances have identified a novel subtype termed HER2-low breast cancer, which is distinguished by low levels of HER2 expression - specifically defined by an immunohistochemistry (IHC) score of 1+ or 2+ without amplification as determined by in situ hybridization (ISH)[3]. This subgroup, previously grouped with HER2-negative breast cancers, has garnered significant attention due to its distinct biological behavior and its potential responsiveness to emerging therapies, particularly antibody-drug conjugates (ADCs)[3].
Differences and similarities between HER2-low and HER2-zero breast cancer
HER2-low breast cancers encompass a heterogeneous group characterized by distinct clinical and molecular features. Although both HER2-low and HER2-zero tumors (those with an IHC score of 0) are categorized as HER2-negative according to traditional classification systems, notable differences exist between them.
In terms of HER2 expression levels, HER2-low tumors exhibit low but measurable HER2 protein expression without gene amplification, whereas HER2-zero tumors show no detectable HER2 expression[4]. Another point of divergence lies in hormone receptor status: HER2-low tumors are frequently hormone receptor-positive (HR+), though they can also appear in triple-negative breast cancers (TNBC). On the other hand, HER2-zero tumors are more commonly associated with TNBC[4].
These distinctions have critical therapeutic implications. The identification of HER2-low status opens new avenues for treatment, particularly with novel antibody-drug conjugates (ADCs) such as trastuzumab deruxtecan, which have demonstrated efficacy in HER2-low breast cancer patients. In contrast, HER2-zero patients do not benefit from HER2-targeted therapies and are instead treated with alternative systemic therapies[4].
At the molecular level, HER2-low tumors may also exhibit unique gene expression profiles that set them apart from HER2-zero tumors. These molecular differences potentially influence tumor behavior and the response to various therapies, underscoring the importance of precise classification in guiding treatment decisions[4].
Advancements in diagnostic techniques
Accurate identification of HER2-low breast cancer is crucial for optimizing therapeutic strategies, yet traditional diagnostic methods such as immunohistochemistry (IHC) and in situ hybridization (ISH) present challenges, including interpretive ambiguity and pre-analytical variability[3]. To address these limitations, recent studies have explored alternative approaches. Notably, a study by Moutafi et al. (2022) compared quantitative immunofluorescence with classical IHC for assessing HER2-low breast cancer, finding that immunofluorescence offers a more precise evaluation of HER2 status[5]. These advancements highlight the potential of integrating molecular diagnostics to enhance the accuracy of breast cancer classification and treatment selection.
The advent of antibody-drug conjugates (ADCs) has significantly transformed the treatment landscape for breast cancer, particularly for patients with HER2-low expression. Trastuzumab deruxtecan, a leading ADC, has demonstrated exceptional efficacy in this subgroup. The DESTINY-Breast04 clinical trial showed that trastuzumab deruxtecan markedly improved both progression-free survival and overall survival compared to standard chemotherapy in HER2-low breast cancer patients[6].
Furthermore, ADCs have shown promise in treating metastatic breast cancer, particularly in cases with central nervous system involvement. A 2023 case series reported successful treatment of eight patients with metastatic HER2-positive breast cancer and leptomeningeal metastases using trastuzumab deruxtecan[7]. These findings suggest that ADCs may provide therapeutic benefits even in advanced metastatic disease, underscoring the potential for broader application of ADCs in HER2-low breast cancer patients.
Importance of liquid biopsy and RNA-based technologies
Advancements in diagnostic technologies are critical for capturing the subtle variations in HER2 expression levels, particularly in the context of HER2-low breast cancer. Emerging techniques, such as liquid biopsy and RNA bead-based assays, provide non-invasive and highly sensitive methods for detecting HER2 expression and other biomarkers in body fluids[8]. These innovative approaches have the potential to significantly improve the accuracy of HER2-low breast cancer identification, thereby enabling more personalized treatment strategies and leading to improved therapeutic outcomes.
This review emphasizes the importance of incorporating cutting-edge technologies, including liquid biopsy and RNA bead-based assays, into the diagnostic framework for breast cancer patients. By enhancing the precision and reliability of molecular classification, especially in identifying HER2-low subtypes, these methods can help better stratify patients for targeted therapies. In doing so, they offer the promise of more tailored treatments and improved outcomes for individuals with breast cancer.
THE SPECTRUM OF HER2 TESTING
The clinical significance of HER2 testing lies in its ability to accurately select and stratify breast cancer patients for treatment with HER2-targeted therapies. These therapies include monoclonal antibodies such as trastuzumab and pertuzumab, tyrosine kinase inhibitors like lapatinib, as well as antibody-drug conjugates (ADCs) like trastuzumab emtansine and trastuzumab deruxtecan, all of which have demonstrated effectiveness in specific patient populations[9,10]. This stratification plays a crucial role in advancing personalized and precision medicine in breast cancer care.
Current standard methods: IHC and FISH
Currently, immunohistochemistry (IHC) serves as the primary screening method for detecting HER2 protein overexpression. In many cases, IHC is combined with fluorescence in situ hybridization (FISH) to assess HER2 gene amplification, following the 2018 updated guidelines issued by the American Society of Clinical Oncology/College of American Pathologists (ASCO/CAP)[11,12].
Challenges with traditional methods
Previous recommendations for HER2 classification often lacked clarity, leading to challenges in clinical decision making, particularly in cases with equivocal results. This ambiguity may have contributed to false-negative findings, which in turn prevented some patients from receiving appropriate HER2-targeted therapies[12]. The ongoing need for more precise and reliable diagnostic tools is evident, as these advancements are critical for improving treatment outcomes and ensuring that patients receive the most effective therapies.
In the realm of breast cancer diagnostics, immunohistochemistry (IHC) is a specialized staining technique utilized to analyze formalin-fixed, paraffin-embedded (FFPE) and fresh frozen tissues obtained from surgical biopsies. This method is primarily employed to determine whether breast cancer cells express HER2 receptors and/or hormone receptors (HR) on their surfaces[13]. The primary objective of IHC is to differentiate between patients with HER2-positive and HER2-negative breast cancer[13].
Fluorescence in situ hybridization (FISH) complements IHC by employing differentially labeled fluorescent probes that target the HER2 gene locus on the long arm of chromosome 17 at 17q12, as well as the centromere of chromosome 17, which is assessed using a chromosome enumeration probe (CEP17). This technique can also evaluate the average HER2 copy number through single-probe analysis, which detects extra copies of the HER2/neu gene within cancer cells[14]. Notably, HER2 protein overexpression occurs in approximately 15%-20% of all diagnosed breast cancer cases, identifying these tumors as HER2-positive. This designation is associated with more aggressive disease progression, poorer prognosis, and shorter overall survival rates[15].
The diagnostic importance of HER2 positivity has evolved over time, becoming more robust and precisely defined. Beyond serving as a prognostic marker, HER2 expression patterns now provide predictive value, indicating potential responses to targeted therapies[16]. According to the latest 2018 ASCO/CAP guidelines, HER2 expression in breast cancer is categorized using semi-quantitative criteria: negative (0 and 1+), equivocal (2+), and positive (3+) based on IHC results. Furthermore, ISH classifies tumors as negative (Group 1), equivocal (Groups 2, 3, and 4), or amplified (positive, Group 5)[11,17]. Evidence-based recommendations suggest that approximately 10%-20% of breast cancers are classified as HER2-positive, defined by IHC scores of 3+ or 2+/ISH+[12,18]. For instance, a score of 3+ indicates strong positivity in IHC, characterized by complete and intense circumferential membranous staining in over 10% of tumor cells. In contrast, positive results in ISH are identified by a dual-probe HER2/CEP17 ratio of 2.0 or greater, along with an average HER2 copy number of at least 4.0 signals per cell, or a single-probe average HER2 copy number of at least 6.0 signals per cell. If the dual-probe HER2/CEP17 ratio is less than 2.0, but the average HER2 copy number reaches or exceeds 6.0 signals per cell, this may indicate HER2 overexpression[12,15].
It is essential to recognize that these classifications differ from the majority of breast cancers, which typically exhibit heterogeneous levels of HER2 expression and do not qualify as HER2-positive. Scoring can be heavily influenced by various factors, including sample selection and collection, which contribute to tissue heterogeneity, artifact production, and other pre-analytical variables, such as the type and duration of fixation agents. Approximately 60% of patients within the HER2-negative subgroup show detectable yet modest levels of HER2 expression, as reflected in IHC-stained slides with scores of 0, 1+, and 2+/ISH-, which are clinically categorized as HER2-negative[13,17].
In the classification for HER2-negative status, testing through IHC and/or ISH typically does not require confirmation by alternative assays. Conversely, cases deemed HER2-uncertain based on IHC or ISH results must be evaluated using a secondary HER2 testing method[12,19].
Determining which method - either IHC or ISH - is superior for establishing an optimal HER2 testing algorithm remains an area of ongoing investigation. The classification of HER2 expression has notably evolved, underscoring the need for reliable standardization processes and clear interpretative criteria[20]. Despite the strong correlation between HER2 protein overexpression as determined by IHC and gene amplification via FISH in archival breast cancer tissue samples, challenges persist. These include higher failure rates and greater resource demands for FISH, which requires expensive fluorescence microscopy and reagents, compared to IHC, which is also influenced by antibody selection, pre-analytical standardization, and variability in interpretation among pathologists[21,22].
Microarray and NGS analysis
The importance of predicting tumor response to therapy in HER2-positive breast cancer is increasing significantly. To achieve this, a key focus in molecular and clinical diagnostics is the differentiation of the five main intrinsic subtypes based on gene expression patterns using DNA microarrays: luminal A, luminal B, HER2-overexpressing or HER2-enriched (HER2-E), basal-like, and normal breast-like[23-25]. Generally, the discrimination of two intrinsic subtypes with genomic analysis in HR+/HER2+ breast cancer patients is most identified: Luminal B and HER2-E[26,27], with the latter associated with high expression of HER2-related and high proliferation genes such as ERBB2/HER2. For example, HER2-positive cases have a higher frequency of the HER2-E subtype, which can also be found in HER2-negative subtypes, both in HR+ and HR− expression profiles, and is characterized by moderate expression of luminal-related proteins and genes (e.g., ESR1, FGFR4, FOXA1), as opposed to reduced expression of basal-related markers (e.g., cytokeratins 5 and 6)[23,28].
The first consideration came from a large number of studies reporting a strong correlation between HER2 mRNA levels and the HER2 gene, suggesting RNA evaluation as a useful option for clinical validation of HER2 status[29]. Over a decade ago, attention shifted to molecular breast cancer signatures as specific biomarkers due to the use of gene-expression-based assays, not limited to histopathological and cytogenetic tests. The introduction of commercial molecular platforms such as Prediction Analysis of Microarray 50 (PAM50, adapted to the NanoString method), Oncotype DX, and MammaTyper assays (qRT-PCR technology) provides comprehensive subtype details based on the expression patterns of multiple genes. Each platform is associated with specific relapse-free survival prognoses and predictions of response to chemotherapy, enhancing intrinsic classification[30,31].
Thus, the aim was to extend the evaluation of HER2 mRNA testing by real-time quantitative PCR (qPCR) and try to combine HER2 DNA amplification and mRNA expression in breast cancer FFPE tissues. Although the idea was valid and interesting, there were discrepancies related to the expected degradation of nucleic acids with amplification bias that could increase unreliable positive results[32,33]. The preliminary purpose was based on the fact that qPCR approaches are considered alternative methods to reduce inconsistent results of IHC. In this regard, the possible concordance between HER2 status determined by IHC and FISH assays was evaluated and compared with results from the Oncotype DX qPCR test. The demonstration of a false-negative rate for Oncotype DX qPCR for HER2 assessment was higher than 50%[18,34], confirming that the uncertainty or possible negativity of qPCR in HER2-positive patients is a problem that has not yet been resolved.
Comparing expression-based assays with those previously based on pathological criteria, it has been observed that a high concordance rate could not be established in a large part of these research findings[25,35-39]. To overcome the challenges faced in standard molecular detection for HER2, a novel in situ mRNA method called "RNAscope" has been developed. RNAscope provides results with nearly 100% accuracy compared to FISH, unlike qPCR, which has shown unreliable concordance rates with FISH and IHC in FFPE breast cancer tissues, particularly in cases of intratumor heterogeneity or equivocal results[40].
Since the intratumor heterogeneity of HER2-positive tumors is described in approximately 35%-45% of all cases, it remains important to address and improve several affecting conditions such as variabilities in HER2 expression, molecular composition of HER2+ subtypes, and different genetic alterations[41-43]. A possible solution to discriminate HER2 somatic mutations and variant allele frequencies can be provided by the detection of circulating tumor DNA (ctDNA) assessed in peripheral blood. It has been demonstrated that HER2-positive cases had a higher rate of these genetic abnormalities than HER2-negative cases, suggesting a possible relation to HER2 gene copy number gain[44,45].
Regarding liquid biopsy, a PCR-based protocol that quantifies HER2 transcript levels is provided by the Droplet Digital PCR System (ddPCR), which aims to partition nucleic acid samples into thousands of nanoliter-sized droplets and allows PCR amplification to occur within each droplet[46-48]. The advent of this method has facilitated the interpretation of two important issues: intratumor heterogeneity and equivocal cases. Not by chance, ddPCR assays could distinguish IHC and FISH uncertain results and classify them as HER2-negative compared to those HER2-positive cases[49].
From a genomic standpoint, there are a large number of alterations, particularly those that belong to
In the spectrum of HER2-positive primary tumors, significant importance is attributed to two common mutations, K753E and L755S, due to their association with drug resistance mechanisms. These genetic alterations hyperactivate both MAPK and PI3K/AKT pathways (20%-40%), leading to HER2 resistance to trastuzumab and tyrosine kinase inhibitors (TKIs)[54]. Specifically, the upregulation of the PI3K/Akt/mTOR pathway is linked to PTEN loss with an increase in PI3K activation[55]. Clinically, it has been demonstrated that PTEN loss correlates with poorer disease-free survival and overall survival in adjuvant anti-HER2 treatment trials in HER2-amplified breast cancer patients[56,57].
NGS panels are commonly used, as previously reported, to detect actionable copy number variants and somatic mutations for targeted therapy and attribute clinical implications. In this specific case, different studies have demonstrated that the assessment of HER2 amplification status by NGS yielded concordant results with FISH. More interestingly, NGS also allows the identification of HER2 amplification in invasive breast carcinoma in cases of negative HER2 by IHC that may not be detected with the current HER2 IHC-first approach. For research purposes, the use of NGS, particularly RNA sequencing (RNA-seq) for gene expression analysis, is taking over and is promptly replacing qRT-PCR in terms of flexibility, sensitivity, and accuracy[58,59]. Despite having many advantages, sometimes it might not be sufficient for routine diagnostic use due to intensive labor and excessive costs. Furthermore, it is important to consider that analyzing high-throughput gene expression data requires several bioinformatic algorithms, which are not always readily available in molecular diagnostics, including those for breast cancer[60].
Thus, the definition of phenotypic classification of breast cancer is evolving as a result of a wide spectrum of gene signatures that are establishing a new biological classification system for breast cancer patients. High-throughput technologies not only allow for the classification of intrinsic molecular subtypes but also help establish and construct clinical prediction models to estimate prognosis and predict the pharmacological response to chemotherapy and HER2-targeted drugs[61,62]. These molecular changes are followed by remarkable improvements in the pathological diagnostic era, which aims for a prognostic classification of breast cancer and deserves further in-depth investigations in clinical settings.
DNA and RNA multiplex assays
Since new promising drugs are about to gain approval to broaden the horizon of HER2-positive breast cancer, the need for a gold standard for HER2 screening tests - as novel, quick, and multiplex assays by RNA-based methods that complement IHC and DNA FISH analysis and accurately classify breast cancer into the different molecular subtypes - remains a priority. Thus, the big challenge to address this clinico-pathological issue and resolve the HER2 status and redefine the complexity of HER2 equivocal results is not far away[18,63]. The multiplexing assay platform takes advantage of multiple targets from the same tissue material, allowing the collection of a greater amount of molecular information for a better classification model with high predictive accuracy and sensitivity compared to single-plex molecular tests [Table 1][64-66].
Feature | Advantage |
High throughput | Reduced cost, labor, and time |
Objective method | Absolute quantification not subjected to interpretation |
High accuracy for multiple targets | Multiple targets quantification allows fast diagnosis; high accuracy requires minimal sample material |
Optimized sampling process | Low specialized facility and human resources |
Great attention has been paid to the different magnetic beads for multiplexed sorting in microfluidic systems in order to isolate cancer-specific exosomes, the major classes of extracellular vesicles (EVs). EVs are produced and released by cancer cells into the surrounding microenvironment. It is well-known that their functions, as well as inter-cellular communication, can influence cell proliferation, motility, and survival by enhancing metastatic activity[67]. Consequently, EVs are expected to be detected in bodily fluids at the early stages of multiple cancers. EVs include several types of proteins, such as surface receptors, signaling proteins, metabolites, extracellular matrix, and RNA-binding proteins. In addition to the aforementioned proteins, EVs contain microRNAs (miRNAs), long non-coding RNAs (lncRNAs), and fragments of genomic DNA[68].
In this context, microfluidic applications based on EV profiling have surpassed the limitations of traditional methods, ensuring reduced processing times and low-input samples with maximum genomic DNA or RNA yields, thus improving the efficiency and sensitivity of the method and the selectivity of the device to detect and capture EV populations, such as tumor-derived EV populations[69]. Several capture chambers are used to facilitate the detection of a single sample by multiple analyses. Technically, this approach is based on a detectable label (fluorophore or dye) attached to a fraction, enhancing the binding specificity for EVs via avidin or polymer. Detection is achieved using primary and secondary antibodies bound to EVs and fluorophores, respectively. Basically, the method is built on an enzyme-linked immunosorbent assay (ELISA) that relies on specific antibodies to bind the target antigen and a detection system to indicate the presence and quantity of antigen binding in bodily fluids[70,71].
Consequently, the analytical process offers valuable information from surface marker expression that may be obtained by measuring such analytes (EV species) present in each chamber. In this way, these acquired measurements are then subsequently processed starting from downstream applications, including a lysis chamber for lysing the exosomes and nucleic acids and/or proteomic profiling[65,72-74]. Beyond bodily fluids, RNA can be optimized from archival FFPE sections, with main challenges that need to be addressed, including RNA degradation and variability in the processing and handling of tissues and fluids[75,76].
A combined approach of branched DNA (bDNA) technology and multi-analyte magnetic beads allows for the detection and quantification of multiple RNA targets simultaneously, overcoming issues related to poor accuracy of gene expression studies. Briefly, this assay works on the hybridization of specific probes for target-specific RNA quantitation with signal amplification production, instead of using target RNA. Particularly, it is based on labeled DNA microspheres that are able to capture specific RNA molecules. DNA amplification releases a signal when hybridization to the tails of the label extenders occurs. In this way, it is possible to quantify multiple target-specific RNAs in a single sample. Detection methods generally used include flow cytometry, fluorescent-dyed microspheres (beads), and digital signal processors (DSP)[77,78].
Furthermore, it must be added that the use of these detection probes not only facilitates the capture but also ensures the recognition of short sequences, aiming to capture short fragments of target RNA with increased specificity. Additionally, the potential of this strategy is linked to the fact that a low amount of RNA is obtained from RNA extraction and purification processes[79].
Moving toward the clinical field, the determination of HER2 status extends to novel applications such as bead-based RNA assay technology to analyze gene expression profiles directly in tissue and cell lysates, such as microdissected material, exosomes, and circulating tumor cells (CTCs). A high level of accuracy and performance is certainly key to adopting the best therapeutic decisions for breast cancer patients in order to perform early detection of cancer recurrence and improve response rates[15]. Compared to other conventional methodologies, a plausible explanation from a biological point of view is that the assessment of HER2 protein expression by IHC and/or gene amplification by FISH is not necessarily related to higher RNA expression levels, since transcriptional and post-transcriptional modifications take over. Thus, the possibility of having a tool available for quickly measuring HER2 and providing digital quantitative analysis could add value for therapeutic response prediction and prognosis, corroborating its clinical significance in diagnostic oncology as a potential first-line test or confirmatory test[63,80].
PERSONALIZED MEDICINE AND LIQUID BIOPSY IN HER2-LOW BREAST CANCER
Personalized cancer medicine represents a revolutionary approach to cancer care, tailoring treatment strategies to the unique characteristics of individual patients and their specific cancer types. This methodology is grounded in a comprehensive understanding of the genetic, molecular, and cellular attributes of a patient’s tumor[81,82]. In the management of breast cancer, particularly HER2-low breast cancer, personalized medicine has become increasingly important, with liquid biopsy emerging as a transformative tool that offers profound insights into tumor biology and enables more personalized treatment strategies.
At the heart of personalized cancer medicine is genomic analysis, which serves as the foundational step in this approach. This process involves sequencing the DNA of cancer cells to identify specific genetic mutations, alterations, and other genomic abnormalities that contribute to the tumor’s growth. The principle underlying personalized cancer medicine is that every patient’s cancer is unique, and thus, its treatment must be equally individualized. Based on the findings from genomic analysis, oncologists can select targeted therapies that specifically address the molecular pathways and genetic mutations driving cancer progression. National data in the United States indicate that the percentage of cancer patients benefiting from targeted therapy increased from 2.73% in 2006 to 7.04% in 2020[83]. These targeted therapies are often more precise and associated with fewer side effects compared to traditional chemotherapy. In recent years, immunotherapy has emerged as a significant component of targeted therapies.
Personalized cancer medicine also takes into account the patient’s immune system, employing immunotherapies designed to leverage the body’s natural immune response to combat cancer. Specific immunotherapies, such as immune checkpoint inhibitors, can be tailored to a patient’s tumor characteristics and immune profile. The introduction of checkpoint inhibitors has had a dramatic impact on cancer treatment; the proportion of cancer patients eligible for checkpoint inhibitor therapy surged from 1.54% in 2011 to 43.63% in 2018[84]. These advancements in immunotherapy and checkpoint inhibition have markedly improved cancer prognosis and survival rates. For instance, in non-small cell lung cancer (NSCLC), the five-year survival rate across all stages increased from 14% to 23.7%. Similarly, in melanoma, immunotherapy has become the first-line treatment option for unresectable or diffuse disease, achieving better survival outcomes compared to standard therapies[85].
The ultimate goal of treatment in personalized cancer medicine is to decrease disease burden and alleviate side effects, often accomplished through combination therapies[86]. In certain cases, a synergistic approach that combines targeted therapies, immunotherapies, and traditional chemotherapy may be employed to enhance treatment effectiveness and overcome resistance mechanisms. Notably, combining targeted therapy with immunotherapy has yielded remarkable results in melanoma patients with the BRAF V600E mutation, leading to an overall survival of 30 months[87].
Another crucial aspect of personalized cancer medicine is the emphasis on monitoring and adaptation. Continuous evaluation of the cancer’s response to treatment is essential for detecting potential relapses and identifying treatment resistance early on. Such monitoring allows for timely adjustments to the treatment plan, optimizing the patient’s care[88].
In this context, liquid biopsy has emerged as a non-invasive diagnostic approach used to detect and analyze cancer-associated biomarkers present in bodily fluids such as blood, urine, milk, or cerebrospinal fluid[89]. Liquid biopsy enables real-time monitoring of tumor dynamics, providing valuable information that can guide personalized treatment strategies, particularly in HER2-low breast cancer.
Circulating tumor DNA analysis
ctDNA consists of small fragments of DNA shed by tumor cells into the bloodstream[90]. Analyzing ctDNA allows for the detection of specific genetic mutations, copy number alterations, and other molecular aberrations associated with HER2-low breast cancer[91]. This is especially crucial because traditional tissue biopsies may not capture the heterogeneity of HER2 expression in these tumors. Liquid biopsy enables real-time monitoring of HER2 expression levels and the identification of actionable mutations, such as PIK3CA mutations, which can inform targeted therapy decisions[92].
Recent studies have demonstrated the utility of ctDNA in tracking tumor dynamics and assessing treatment response in HER2-low breast cancer patients[93]. For instance, ctDNA levels have been correlated with tumor burden and can predict disease progression or response to therapies like novel antibody-drug conjugates (ADCs) designed for low HER2 expression[7]. Moreover, ctDNA analysis can detect emerging resistance mutations, allowing for timely adjustments in therapeutic strategies to improve patient outcomes.
Circulating tumor cells enumeration and characterization
CTCs are cancer cells that have detached from the primary tumor and entered the bloodstream. Analyzing CTCs provides valuable information about tumor heterogeneity and metastatic potential in HER2-low breast cancer[94]. Techniques such as microfluidic devices and immunomagnetic separation have enhanced the sensitivity and specificity of CTC detection.
In HER2-low breast cancer, CTC analysis can reveal changes in HER2 expression levels that may not be apparent in the primary tumor biopsy. For example, some patients may exhibit an increase in HER2 expression in CTCs during disease progression, suggesting a potential shift in tumor phenotype that could be targeted with HER2-directed therapies[94]. Additionally, CTC characterization can help identify other biomarkers, such as hormone receptor status, aiding in the selection of appropriate systemic treatments.
Clinical applications and future perspectives
Implementing liquid biopsy in clinical practice for HER2-low breast cancer holds significant promise. It facilitates early detection of disease recurrence, monitors therapeutic responses, and assesses minimal residual disease without the need for invasive procedures. The integration of ctDNA and CTC analyses can guide clinicians in tailoring treatments, optimizing therapeutic efficacy, and mitigating resistance.
Recent clinical trials have explored the use of liquid biopsy-guided interventions in HER2-low breast cancer patients. For instance, studies have shown that ctDNA dynamics can predict response to ADCs like trastuzumab deruxtecan, allowing for personalized treatment adjustments[7]. Ongoing research aims to further validate the clinical utility of liquid biopsy in this patient population.
Ultimately, the goal of personalized cancer medicine, enhanced by liquid biopsy technologies, is to optimize treatment outcomes, enhance remission rates, and minimize side effects by customizing therapies to the unique characteristics of each patient’s cancer[81,95]. This approach has led to significant advancements in cancer care, contributing to improved survival rates and enhanced quality of life for many patients. However, it is important to acknowledge that the applicability and effectiveness of personalized treatments can vary based on the specific cancer type and genetic alterations involved, underscoring the necessity for thorough evaluations by a healthcare team to determine the most appropriate treatment plan.
CONCLUSION AND FUTURE PERSPECTIVES
The misdiagnosis of HER2 status is a critical issue that profoundly impacts the prognostic and therapeutic outcomes for breast cancer patients. This challenge underscores the urgent need for rigorous quality control measures and the development of highly sensitive and rapid systems for detecting HER2 expression levels. Such advancements are particularly vital for the accurate identification of HER2-low breast cancers, which represent an important and emerging category within the broader HER2-negative spectrum. Enhancing diagnostic techniques to discern the subtle distinctions between HER2-low and HER2-zero tumors is essential, as these differences can significantly influence treatment decisions and ultimately improve patient outcomes [Figure 1].
Figure 1. The image visually represents breast cancer classification based on molecular biology. It highlights different molecular subtypes of breast cancer, such as HER2-positive and hormone receptor-positive tumors. The bottom bar showcases various diagnostic tools, including traditional tissue biopsies, immunohistochemistry, liquid biopsy techniques like ctDNA and CTC analysis, and RNA-based screening methods. The image emphasizes the role of molecular profiling in guiding personalized treatment strategies for breast cancer patients. Image created with Biorender.com.
To address these challenges, we advocate for the integration of advanced diagnostic technologies, particularly liquid biopsy and RNA bead-based assays, which can enhance the sensitivity and precision of HER2 detection. Liquid biopsy, in particular, stands out as a transformative technological advancement, enabling sensitive and accurate assessments of multiple target genes from bodily fluids. This approach holds considerable promise for improving patient survival by addressing three critical aspects of cancer care: multi-cancer early detection, determination of tumor therapeutic response, and monitoring for minimal residual disease. Currently, effective early detection screening methods are not universally available for all cancer types, and monitoring therapeutic response remains a significant challenge due to the limitations inherent in traditional tissue biopsies, which may not provide comprehensive insights into the tumor’s molecular mechanisms. Liquid biopsy offers a non-invasive alternative that facilitates real-time monitoring of tumor dynamics, allowing for timely adjustments to treatment strategies. Furthermore, expanding minimal residual disease monitoring beyond hematological malignancies to include solid tumors such as breast cancer could aid in managing treatment decisions and preventing relapse.
Understanding the biological mechanisms underlying HER2-low expression and its implications for tumor behavior and therapy response is paramount. Ongoing research in this domain will pave the way for the development of tailored therapies that enhance outcomes for patients within this subgroup. The classification of HER2 breast cancer subtypes, coupled with the reduction of subjective interpretation and technical variability, is benefiting from advancements in diagnostic technologies. By implementing these sophisticated techniques into clinical practice, we can significantly enhance the accuracy and efficacy of breast cancer diagnostics and treatment. Ultimately, these innovations promise to contribute to improved patient care and better survival outcomes.
DECLARATIONS
Authors’ contributions
Made substantial contributions to the conception and design of the review: Stingi A, Fabrizio FP
Revision and supervision: d’Alessandro A (d’Alessandro Aldo), Deaconu AF, Mandolesi S, Lombardi M, Liguori G, Pepe G, Marino N, d’Alessandro A (d’Alessandro Alessandro), Giordano A
Availability of data and materials
Not applicable.
Financial support and sponsorship
None.
Conflicts of interest
Giordano A is an Editorial Board member of Journal of Cancer Metastasis and Treatment. while the other authors have declared that they have no conflicts of interest.
Ethical approval and consent to participate
Not applicable.
Consent for publication
Not applicable.
Copyright
© The Author(s) 2024.
REFERENCES
1. Eliyatkın N, Yalçın E, Zengel B, Aktaş S, Vardar E. Molecular classification of breast carcinoma: from traditional, old-fashioned way to a new age, and a new way. J Breast Health 2015;11:59-66.
2. Dunnwald LK, Rossing MA, Li CI. Hormone receptor status, tumor characteristics, and prognosis: a prospective cohort of breast cancer patients. Breast Cancer Res 2007;9:R6.
3. Shirman Y, Lubovsky S, Shai A. HER2-low breast cancer: current landscape and future prospects. Breast Cancer 2023;15:605-16.
4. Ergun Y, Ucar G, Akagunduz B. Comparison of HER2-zero and HER2-low in terms of clinicopathological factors and survival in early-stage breast cancer: a systematic review and meta-analysis. Cancer Treat Rev 2023;115:102538.
5. Moutafi M, Robbins CJ, Yaghoobi V, et al. Quantitative measurement of HER2 expression to subclassify ERBB2 unamplified breast cancer. Lab Invest 2022;102:1101-8.
6. Modi S, Jacot W, Yamashita T, et al. Trastuzumab deruxtecan in previously treated HER2-low advanced breast cancer. N Engl J Med 2022;387:9-20.
7. Rogawski D, Cao T, Ma Q, et al. Durable responses to trastuzumab deruxtecan in patients with leptomeningeal metastases from breast cancer with variable HER2 expression. J Neurooncol 2024;170:209-17.
8. Nikanjam M, Kato S, Kurzrock R. Liquid biopsy: current technology and clinical applications. J Hematol Oncol 2022;15:131.
9. Scaltriti M, Nuciforo P, Bradbury I, et al. High HER2 expression correlates with response to the combination of lapatinib and trastuzumab. Clin Cancer Res 2015;21:569-76.
10. Swain SM, Shastry M, Hamilton E. Targeting HER2-positive breast cancer: advances and future directions. Nat Rev Drug Discov 2023;22:101-26.
11. Wolff AC, Hammond MEH, Allison KH, et al. Human epidermal growth factor receptor 2 testing in breast cancer: American society of clinical oncology/college of American pathologists clinical practice guideline focused update. J Clin Oncol 2018;36:2105-22.
12. Early Breast Cancer Trialists’ Collaborative group (EBCTCG). Trastuzumab for early-stage, HER2-positive breast cancer: a meta-analysis of 13 864 women in seven randomised trials. Lancet Oncol 2021;22:1139-50.
13. Iqbal N, Iqbal N. Human epidermal growth factor receptor 2 (HER2) in cancers: overexpression and therapeutic implications. Mol Biol Int 2014;2014:852748.
14. Geiersbach KB, Sill DR, Meyer RG, et al. HER2 testing for breast cancer in the genomics laboratory: a sea change for fluorescence in situ hybridization. Arch Pathol Lab Med 2021;145:883-6.
15. Schettini F, Chic N, Brasó-Maristany F, et al. Clinical, pathological, and PAM50 gene expression features of HER2-low breast cancer. NPJ Breast Cancer 2021;7:1.
16. Marchiò C, Annaratone L, Marques A, Casorzo L, Berrino E, Sapino A. Evolving concepts in HER2 evaluation in breast cancer: Heterogeneity, HER2-low carcinomas and beyond. Semin Cancer Biol 2021;72:123-35.
17. Ahn S, Woo JW, Lee K, Park SY. HER2 status in breast cancer: changes in guidelines and complicating factors for interpretation. J Pathol Transl Med 2020;54:34-44.
18. Fernandez AI, Liu M, Bellizzi A, et al. Examination of low ERBB2 protein expression in breast cancer tissue. JAMA Oncol 2022;8:1-4.
19. Guerini-Rocco E, Venetis K, Cursano G, et al. Standardized molecular pathology workflow for ctDNA-based ESR1 testing in HR+/HER2- metastatic breast cancer. Crit Rev Oncol Hematol 2024;201:104427.
20. Couturier J, Vincent-Salomon A, Nicolas A, et al. Strong correlation between results of fluorescent in situ hybridization and immunohistochemistry for the assessment of the ERBB2 (HER-2/neu) gene status in breast carcinoma. Mod Pathol 2000;13:1238-43.
21. Lebeau A, Deimling D, Kaltz C, et al. Her-2/neu analysis in archival tissue samples of human breast cancer: comparison of immunohistochemistry and fluorescence in situ hybridization. J Clin Oncol 2001;19:354-63.
22. Godoy-Ortiz A, Sanchez-Muñoz A, Chica Parrado MR, et al. Deciphering HER2 breast cancer disease: biological and clinical implications. Front Oncol 2019;9:1124.
23. Sørlie T, Perou CM, Tibshirani R, et al. Gene expression patterns of breast carcinomas distinguish tumor subclasses with clinical implications. Proc Natl Acad Sci USA 2001;98:10869-74.
24. Perou CM, Sørlie T, Eisen MB, et al. Molecular portraits of human breast tumours. Nature 2000;406:747-52.
25. The Cancer Genome Atlas Network. Comprehensive molecular portraits of human breast tumours. Nature 2012;490:61-70.
26. Prat A, Pineda E, Adamo B, et al. Clinical implications of the intrinsic molecular subtypes of breast cancer. Breast 2015;24 Suppl 2:S26-35.
27. Lesurf R, Griffith OL, Griffith M, et al. Genomic characterization of HER2-positive breast cancer and response to neoadjuvant trastuzumab and chemotherapy-results from the ACOSOG Z1041 (Alliance) trial. Ann Oncol 2017;28:1070-7.
28. Benöhr P, Henkel V, Speer R, et al. Her-2/neu expression in breast cancer - A comparison of different diagnostic methods. Anticancer Res 2005;25:1895-900.
29. Paik S, Shak S, Tang G, et al. A multigene assay to predict recurrence of tamoxifen-treated, node-negative breast cancer. N Engl J Med 2004;351:2817-26.
30. van’t Veer LJ, Dai H, van de Vijver MJ, et al. Gene expression profiling predicts clinical outcome of breast cancer. Nature 2002;415:530-6.
31. Gjerdrum LM, Sorensen BS, Kjeldsen E, Sorensen FB, Nexo E, Hamilton-Dutoit S. Real-time quantitative PCR of microdissected paraffin-embedded breast carcinoma: an alternative method for HER-2/neu analysis. J Mol Diagn 2004;6:42-51.
32. Bastien RR, Rodríguez-Lescure Á, Ebbert MT, et al. PAM50 breast cancer subtyping by RT-qPCR and concordance with standard clinical molecular markers. BMC Med Genomics 2012;5:44.
33. Chia SK, Bramwell VH, Tu D, et al. A 50-gene intrinsic subtype classifier for prognosis and prediction of benefit from adjuvant tamoxifen. Clin Cancer Res 2012;18:4465-72.
34. Dowsett M, Sestak I, Lopez-Knowles E, et al. Comparison of PAM50 risk of recurrence score with oncotype DX and IHC4 for predicting risk of distant recurrence after endocrine therapy. J Clin Oncol 2013;31:2783-90.
35. Gnant M, Filipits M, Greil R, et al. Predicting distant recurrence in receptor-positive breast cancer patients with limited clinicopathological risk: using the PAM50 risk of Recurrence score in 1478 postmenopausal patients of the ABCSG-8 trial treated with adjuvant endocrine therapy alone. Ann Oncol 2014;25:339-45.
36. Prat A, Bianchini G, Thomas M, et al. Research-based PAM50 subtype predictor identifies higher responses and improved survival outcomes in HER2-positive breast cancer in the NOAH study. Clin Cancer Res 2014;20:511-21.
37. Wang F, Flanagan J, Su N, et al. RNAscope: a novel in situ RNA analysis platform for formalin-fixed, paraffin-embedded tissues. J Mol Diagn 2012;14:22-9.
38. Allison KH, Dintzis SM, Schmidt RA. Frequency of HER2 heterogeneity by fluorescence in situ hybridization according to CAP expert panel recommendations: time for a new look at how to report heterogeneity. Am J Clin Pathol 2011;136:864-71.
39. Bartlett AI, Starcyznski J, Robson T, et al. Heterogeneous HER2 gene amplification: impact on patient outcome and a clinically relevant definition. Am J Clin Pathol 2011;136:266-74.
40. Hanna WM, Rüschoff J, Bilous M, et al. HER2 in situ hybridization in breast cancer: clinical implications of polysomy 17 and genetic heterogeneity. Mod Pathol 2014;27:4-18.
41. Lee K, Lee J, Choi J, et al. Genomic analysis of plasma circulating tumor DNA in patients with heavily pretreated HER2 + metastatic breast cancer. Sci Rep 2023;13:9928.
42. Tierno D, Grassi G, Zanconati F, Bortul M, Scaggiante B. An overview of circulating cell-free nucleic acids in diagnosis and prognosis of triple-negative breast cancer. Int J Mol Sci 2023;24:1799.
44. Zhu Y, Lu D, Lira ME, et al. Droplet digital polymerase chain reaction detection of HER2 amplification in formalin fixed paraffin embedded breast and gastric carcinoma samples. Exp Mol Pathol 2016;100:287-93.
45. Ferrari A, Vincent-Salomon A, Pivot X, et al. A whole-genome sequence and transcriptome perspective on HER2-positive breast cancers. Nat Commun 2016;7:12222.
46. Zhao S, Liu XY, Jin X, et al. Molecular portraits and trastuzumab responsiveness of estrogen receptor-positive, progesterone receptor-positive, and HER2-positive breast cancer. Theranostics 2019;9:4935-45.
47. Li K, Liao N, Chen B, et al. Genetic mutation profile of Chinese HER2-positive breast cancers and genetic predictors of responses to Neoadjuvant anti-HER2 therapy. Breast Cancer Res Treat 2020;183:321-32.
48. Omarini C, Bettelli S, Manfredini S, et al. Modulation of mutational landscape in HER2-positive breast cancer after neoadjuvant chemotherapy. Transl Oncol 2020;13:100794.
49. Li J, Xiao Q, Bao Y, et al. HER2-L755S mutation induces hyperactive MAPK and PI3K-mTOR signaling, leading to resistance to HER2 tyrosine kinase inhibitor treatment. Cell Cycle 2019;18:1513-22.
50. Georgescu MM. PTEN tumor suppressor network in PI3K-Akt pathway control. Genes Cancer 2010;1:1170-7.
51. Piccart M, Procter M, Fumagalli D, et al. Adjuvant pertuzumab and trastuzumab in early HER2-positive breast cancer in the APHINITY trial: 6 years’ follow-up. J Clin Oncol 2021;39:1448-57.
52. Stern HM, Gardner H, Burzykowski T, et al. PTEN loss is associated with worse outcome in HER2-amplified breast cancer patients but is not associated with trastuzumab resistance. Clin Cancer Res 2015;21:2065-74.
53. Kukurba KR, Montgomery SB. RNA sequencing and analysis. Cold Spring Harb Protoc 2015;2015:951-69.
54. Lee HB, Lee SB, Kim M, et al. Development and validation of a next-generation sequencing-based multigene assay to predict the prognosis of estrogen receptor-positive, HER2-negative breast cancer. Clin Cancer Res 2020;26:6513-22.
55. Morsberger L, Pallavajjala A, Long P, et al. HER2 amplification by next-generation sequencing to identify HER2-positive invasive breast cancer with negative HER2 immunohistochemistry. Cancer Cell Int 2022;22:350.
56. Mittempergher L, Delahaye LJMJ, Witteveen AT, et al. MammaPrint and BluePrint molecular diagnostics using targeted RNA next-generation sequencing technology. J Mol Diagn 2019;21:808-23.
57. Pfarr N, Penzel R, Endris V, et al. Targeted next-generation sequencing enables reliable detection of HER2 (ERBB2) status in breast cancer and provides ancillary information of clinical relevance. Genes Chromosomes Cancer 2017;56:255-65.
58. Sajjadi E, Guerini-Rocco E, De Camilli E, et al. Pathological identification of HER2-low breast cancer: tips, tricks, and troubleshooting for the optimal test. Front Mol Biosci 2023;10:1176309.
59. Baldacchino S, Saliba C, Scerri J, Scerri C, Grech G. Optimization of a multiplex RNA-based expression assay using breast cancer archival material. J Vis Exp 2018;138:57148.
60. Tighe PJ, Ryder RR, Todd I, Fairclough LC. ELISA in the multiplex era: potentials and pitfalls. Proteomics Clin Appl 2015;9:406-22.
61. Wharton KA Jr, Wood D, Manesse M, Maclean KH, Leiss F, Zuraw A. Tissue multiplex analyte detection in anatomic pathology - pathways to clinical implementation. Front Mol Biosci 2021;8:672531.
62. Clancy JW, D'Souza-Schorey C. Tumor-derived extracellular vesicles: multifunctional entities in the tumor microenvironment. Annu Rev Pathol 2023;18:205-29.
63. Zhao H, Achreja A, Iessi E, et al. The key role of extracellular vesicles in the metastatic process. Biochim Biophys Acta Rev Cancer 2018;1869:64-77.
64. Contreras-Naranjo JC, Wu HJ, Ugaz VM. Microfluidics for exosome isolation and analysis: enabling liquid biopsy for personalized medicine. Lab Chip 2017;17:3558-77.
65. Alhajj M, Zubair M, Farhana A. Enzyme linked immunosorbent assay. FL: StatPearls Publishing, 2023. Available from: https://www.ncbi.nlm.nih.gov/books/NBK555922/ [Last accessed on 29 Oct 2024]
66. Im K, Mareninov S, Diaz MFP, Yong WH. An introduction to performing immunofluorescence staining. Methods Mol Biol 2019;1897:299-311.
67. El-Deiry WS, Goldberg RM, Lenz HJ, et al. The current state of molecular testing in the treatment of patients with solid tumors, 2019. CA Cancer J Clin 2019;69:305-43.
68. Im H, Shao H, Park YI, et al. Label-free detection and molecular profiling of exosomes with a nano-plasmonic sensor. Nat Biotechnol 2014;32:490-5.
69. Lindeman NI, Cagle PT, Aisner DL, et al. Updated molecular testing guideline for the selection of lung cancer patients for treatment with targeted tyrosine kinase inhibitors: guideline from the college of American pathologists, the international association for the study of lung cancer, and the association for molecular pathology. J Mol Diagn 2018;20:129-59.
70. Abrahamsen HN, Steiniche T, Nexo E, Hamilton-Dutoit SJ, Sorensen BS. Towards quantitative mRNA analysis in paraffin-embedded tissues using real-time reverse transcriptase-polymerase chain reaction: a methodological study on lymph nodes from melanoma patients. J Mol Diagn 2003;5:34-41.
71. Macabeo-Ong M, Ginzinger DG, Dekker N, et al. Effect of duration of fixation on quantitative reverse transcription polymerase chain reaction analyses. Mod Pathol 2002;15:979-87.
72. Dunbar SA. Nucleic acid sample preparation techniques for bead-based suspension arrays. Methods 2023;219:22-9.
73. Flagella M, Bui S, Zheng Z, et al. A multiplex branched DNA assay for parallel quantitative gene expression profiling. Anal Biochem 2006;352:50-60.
74. Knudsen BS, Allen AN, McLerran DF, et al. Evaluation of the branched-chain DNA assay for measurement of RNA in formalin-fixed tissues. J Mol Diagn 2008;10:169-76.
75. Duenwald S, Zhou M, Wang Y, et al. Development of a microarray platform for FFPET profiling: application to the classification of human tumors. J Transl Med 2009;7:65.
76. Cisneros-Villanueva M, Hidalgo-Pérez L, Rios-Romero M, et al. Cell-free DNA analysis in current cancer clinical trials: a review. Br J Cancer 2022;126:391-400.
77. Connal S, Cameron JM, Sala A, et al. Liquid biopsies: the future of cancer early detection. J Transl Med 2023;21:118.
78. Hoeben A, Joosten EAJ, van den Beuken-van Everdingen MHJ. Personalized medicine: recent progress in cancer therapy. Cancers 2021;13:242.
79. Haslam A, Kim MS, Prasad V. Updated estimates of eligibility for and response to genome-targeted oncology drugs among US cancer patients, 2006-2020. Ann Oncol 2021;32:926-32.
80. Haslam A, Prasad V. Estimation of the percentage of US patients with cancer who are eligible for and respond to checkpoint inhibitor immunotherapy drugs. JAMA Netw Open 2019;2:e192535.
81. Lamba N, Ott PA, Iorgulescu JB. Use of first-line immune checkpoint inhibitors and association with overall survival among patients with metastatic melanoma in the anti-PD-1 era. JAMA Netw Open 2022;5:e2225459.
82. Bayat Mokhtari R, Homayouni TS, Baluch N, et al. Combination therapy in combating cancer. Oncotarget 2017;8:38022-43.
83. Yu C, Liu X, Yang J, et al. Combination of immunotherapy with targeted therapy: theory and practice in metastatic melanoma. Front Immunol 2019;10:990.
84. Zhu L, Xu R, Yang L, et al. Minimal residual disease (MRD) detection in solid tumors using circulating tumor DNA: a systematic review. Front Genet 2023;14:1172108.
85. Gambardella V, Tarazona N, Cejalvo JM, et al. Personalized medicine: recent progress in cancer therapy. Cancers 2020;12:1009.
86. Zhang W. Advances in cancer early diagnosis with liquid biopsy-based approaches. J Cancer Metastasis Treat 2021;7:22.
87. Mazzitelli C, Santini D, Corradini AG, et al. Liquid biopsy in the management of breast cancer patients: where are we now and where are we going. Diagnostics 2023;13:1241.
88. Palacín-Aliana I, García-Romero N, Asensi-Puig A, Carrión-Navarro J, González-Rumayor V, Ayuso-Sacido Á. Clinical utility of liquid biopsy-based actionable mutations detected via ddPCR. Biomedicines 2021;9:906.
89. Baev V, Koppers-Lalic D, Costa-Silva B. Liquid biopsy: current status and future perspectives. Cancers 2023;15:3205.
90. Takahashi RU, Prieto-Vila M, Hironaka A, Ochiya T. The role of extracellular vesicle microRNAs in cancer biology. Clin Chem Lab Med 2017;55:648-56.
91. Lone SN, Nisar S, Masoodi T, et al. Liquid biopsy: a step closer to transform diagnosis, prognosis and future of cancer treatments. Mol Cancer 2022;21:79.
92. Moding EJ, Nabet BY, Alizadeh AA, Diehn M. Detecting liquid remnants of solid tumors: circulating tumor DNA minimal residual disease. Cancer Discov 2021;11:2968-86.
93. Kilgour E, Rothwell DG, Brady G, Dive C. Liquid biopsy-based biomarkers of treatment response and resistance. Cancer Cell 2020;37:485-95.
94. Berger MF, Mardis ER. The emerging clinical relevance of genomics in cancer medicine. Nat Rev Clin Oncol 2018;15:353-65.
Cite This Article

How to Cite
d’Alessandro, A, Deaconu, A. F.; Mandolesi, S.; Fabrizio, F. P.; Lombardi, M.; Liguori, G.; Pepe, G.; Marino, N.; Stingi, A.; d’Alessandro, A.; Giordano, A. Liquid biopsy-based technologies: a promising tool for biomarker identification in her2-low breast cancer patients for improved therapeutic outcomes. J. Cancer. Metastasis. Treat. 2024, 10, 29. http://dx.doi.org/10.20517/2394-4722.2024.63
Download Citation
Export Citation File:
Type of Import
Tips on Downloading Citation
Citation Manager File Format
Type of Import
Direct Import: When the Direct Import option is selected (the default state), a dialogue box will give you the option to Save or Open the downloaded citation data. Choosing Open will either launch your citation manager or give you a choice of applications with which to use the metadata. The Save option saves the file locally for later use.
Indirect Import: When the Indirect Import option is selected, the metadata is displayed and may be copied and pasted as needed.
About This Article
Copyright
Data & Comments
Data
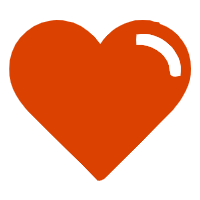
Comments
Comments must be written in English. Spam, offensive content, impersonation, and private information will not be permitted. If any comment is reported and identified as inappropriate content by OAE staff, the comment will be removed without notice. If you have any queries or need any help, please contact us at support@oaepublish.com.