Cell-supplemented autologous fat grafting: a review from bench to bedside
Abstract
Autologous fat grafting is routinely used in plastic and reconstructive surgery to improve contour deformities and appearance. However, retention of fat over time is inconsistent and unpredictable. Cell-assisted lipostransfer (CAL), the practice of using stem cell-containing portions of adipose to enrich fat grafts, has been found to be a promising area of research to increase not only the retention of volume but also increase collagen production, enhance angiogenesis, and direct the overall repair, remodeling, and regeneration of the recipient site. CAL, therefore, has a multitude of clinical applications, ranging from oncologic reconstruction following radiation to facial rejuvenation, among others. In this paper, we provide a comprehensive review of the current state of cell-supplemented fat transfer and discuss our understanding of cellular interactions in enriched fat grafts, techniques to improve the viability of fat, clinical translation of CAL, regulatory oversight, and future directions.
Keywords
INTRODUCTION
Fat grafting has become an increasingly common procedure in the realm of both cosmetic and reconstructive plastic surgery. First described by Neuber et al. in 1893 for the volumetric filling of defects, the procedure has since gone through over a century of development and innovation[1,2]. A vast majority of progress occurred following the advent of liposuction in the late 20th century, which allowed for a more accessible method of obtaining fat with minimal donor site morbidity[3,4]. Further work by surgeons such as Coleman et al. refined both the fat harvest and grafting procedure, with particular emphasis on atraumatic harvest and small aliquot grafting to increase the viability of fat[5].
However, despite the best efforts of improved technology and user experience, consistency in fat grafting results remains elusive, with resorption rates quoted between 30%-70% within the first year[6]. This may be attributed to technical aspects of fat harvest, processing, and injection, as well as the natural heterogeneity of the cell population within the graft itself[7-9]. Methods to increase predictability of results, and specifically volume retention, have therefore been highly sought after. There are several proposed mechanisms for graft survival. First, adipose grafts, like skin grafts, are dependent on nutrient diffusion from the recipient site until neovascularization. The host replacement theory states that host histiocytes would eventually take the place of grafted fat, and the cell survival theory states that a subpopulation of grafted fat is able to survive in the new host environment[10]. In 2001, Zuk et al. identified adult, multipotent progenitor cells within the stroma of the adipose tissue. Subsequent work over the past few decades has deemed the cell survival theory the predominant mechanism, with adipose-derived stem cells (ASCs) contributing substantially to mature adipocytes in grafted fat[11,12]. In addition, the paracrine effect of these cells on the graft and surrounding tissue continues to be uncovered.
The discovery of ASCs opened the door for a variety of adipose-derived therapeutics in regenerative medicine. Among such applications is the concept of supplementing traditional grafts with progenitor cells from the stromal vascular fraction (SVF), or so-called “cell-assisted lipotransfer” (CAL). Cell supplementation has been proposed to improve graft survival or retention, but more recently set the foundation for engineered adipose grafts in several translational applications.
CELLULAR COMPONENTS OF FAT
Adipose tissue is comprised of numerous cell populations, including adipocytes, ASCs, fibroblasts, immune cells, and endothelial cells, among others. A 2009 study by Eto et al. examined the components of human fat tissue, and found that mature adipocytes comprised only a small fraction of aspirated fat, while the majority of cells were termed “vasculature-associated cells” and included ASCs, inflammatory cells, and endothelial cells[13]. The isolation of these latter cells from fat was first described by Rodbell et al. in 1966 and has largely remained the same conceptually - fat harvesting, processing, collagenase digestion, and resuspension to acquire the SVF consisting of ASCs, fibroblasts, vascular progenitor cells, pericytes, and endothelial cells[14,15]. This SVF, when transplanted along with fat grafts, has been shown on a cellular level to actively participate in adipogenesis and angiogenesis[16]. ASCs can further be expanded from the SVF and are able to differentiate into a variety of cell lineages with similar multipotency to bone marrow-derived stem cells[17,18]. The ASC population is activated under hypoxic conditions that predominate the microenvironment of grafted fat. The activation of ASCs contributes to both adipocyte repair through differentiation into adipocytes and integration with surviving grafted fat, as well as angiogenesis via differentiation into endothelial cells[19-22]. Beyond direct cellular and vascular contributions, the paracrine effects of ASCs and the SVF increase the production of collagen and elastin, further promoting surrounding adipogenesis and angiogenesis while reducing fibrosis[23,24].
FAT AND SVF HARVEST TECHNIQUES
The optimal grafting technique to preserve fat viability and minimize adipocyte death is controversial. The traditional Coleman technique emphasized fat harvest using an atraumatic process with a 3-mm cannula attached to a 10-cc syringe, followed by centrifugation and injection of small aliquots of fat into multiple tissue planes[25-27]. However, further research has found suction-assisted liposuction (SAL) and power-assisted liposuction (PAL), as well as alternative processing techniques, as effective ways for obtaining viable fat[28,29]. PAL and ultrasound-assisted liposuction (UAL) have also been found to collect equivalent amounts of ASCs to SAL[30,31]. Additionally, the use of a 2-mm diameter multiperforated microcannula and lower suction pressures have been shown to increase ASC yield in isolation[32-34].
Similarly, with cell-supplementation such as CAL, the optimal technique is yet to be determined. In a standard approach, fat is typically harvested via liposuction to ensure an adequate quantity of ASCs. It is then washed with sterile phosphate-buffered saline (PBS), digested with collagenase, centrifuged, and resuspended[27,35]. Depending on the quantity of ASCs, the sample can then be used directly or expanded in culture to ensure adequate sample size. Several products have come on the market to help facilitate and automate the process of enzymatic isolation. Aronowitz et al. compared four of these products - the Multi-Station (PNC International, Gyeonggi-do, Republic of Korea), the Cha-Station (CHA Biotech, Kangnamgu, Republic of Korea), the Lipokit with MaxStem (Medi-Khan, West Hollywood, Calif.), and the Celution 800/CRS System (Cytori Therapeutics, Inc., San Diego, Calif.) - in their efficiency in isolating SVF in vitro[36]. They found the latter to yield a significantly higher concentration of SVF and endothelial cells, with minimal residual collagenase activity.
However, the safety of enzymatic digestion with the potential for residual collagenase in SVF samples has been questioned, and purely mechanical means of SVF have been described as well[37,38]. Tiryaki et al. describe a three-step process of manual isolation utilizing initial processing through different sizes of microblades, incubation and centrifugation, and resuspension of the SVF pellet after discarding supernatant material[39]. They compared their methods to traditional enzymatic processing, and though the latter had a higher SVF cell concentration, mechanical digestion had equal cell viability and higher stem cell surface markers. Therefore, mechanical digestion may provide a faster and cheaper means of SVF isolation.
Large-scale studies examining the clinical implications of these findings remain limited. Domenis et al. compared the enzymatic Celution and Lipokit systems to the mechanical Fastem (CORIOS Soc. Coop, San Giuliano Milanese MI, Italy) system[40]. While the mechanical system had less potential for multipotency in vitro, there was no difference in clinical fat retention at 12 months when comparing the three methods. A similarly designed study by Gentile et al. focused mostly on clinical outcomes and found the enzymatic Celution systems to be superior in terms of isolating SVF and maintaining volume[41]. A more recent study published by this group prospectively compared fat grafting enhanced with SVF either isolated enzymatically or mechanically (via filtration alone, centrifugation alone, or filtration and centrifugation) and found that filtration was the most efficient mechanical method of isolation leading to the highest fat retention at 1-year follow-up, though this was still superseded by enzymatic isolation[42].
The difference in ASCs from different anatomical fat deposits has also been of recent interest. Di Taranto et al. examined the differences between superficial and deep abdominal adipose tissue from female donors and found the superficial layer of fat had higher ASC concentration and increased multipotency when isolated[43]. Faustini et al., studying SVF concentration differences in males versus females, found the abdomen to be the best fat harvest site in males, whereas they did not find any significant anatomical difference in SVF yield in females from different fat deposits[44]. In contrast, Tsekouras et al. found a significantly higher yield of ASCs from the inner and outer thigh compared to the abdomen, while Grasys et al. found the opposite[45,46]. One of the most comprehensive studies regarding harvest technique and anatomical site of harvest was conducted by Iyyanki et al. in 2015[47]. In this study, fat was harvested from the abdomen, flank, or axilla using liposuction, direct excision or the Coleman technique with or without centrifugation. They found that direct excision led to the highest SVF and ASC concentration while liposuction led to the lowest. They also found significantly higher SVF concentration from the abdomen, though ASC concentration did not differ between anatomical sites. Therefore, there is still conflicting evidence regarding the harvest technique and location in regards to optimizing ASC yield and the donor site should be chosen based on surgeon preference and patient body habitus.
CELL-ASSISTED LIPOTRANSFER
The majority of work on the utility of CAL has focused on increasing fat graft survival. One of the earliest demonstrations of this was carried out in 2006 by Matsumoto et al., who augmented human fat grafts with isolated SVF from the same samples and subsequently transplanted them into immunodeficient mice[48]. They found a 35% increased survival in CAL-augmented fat grafts, as well as increased microvasculature and angiogenesis. One of the first studies in patients was conducted by Yoshimura et al. in cosmetic breast augmentation, who showed a promising retainment of breast volume at 6 to 12 months post-transplantation with SVF-enriched fat[49,50]. Improved graft retention in the breast with CAL has subsequently been shown by several groups, including a prospective trial examining the use of SVF-augmented fat grafting to correct contour defects following breast-conserving therapy (BCT)[51-54]. One of the few randomized controlled trials in CAL fat grafting in the breast was done by Kolle et al. in 2020, which compared fat grafts alone to fat grafts augmented with ex vivo expanded ASCs in comparable cohorts of patients[55]. They found increased retention with ASC augmentation compared to fat grafting alone (80.2% vs. 45.1%), as well as superior subjective outcomes when assessed by blinded observers.
Yoshimura et al. have shown that fat retention in facial fat grafting has an improvement with SVF inclusion and ASC inclusion[56]. In a prospective study, Koh et al. found a resorption of 46.81% with fat grafting alone compared to 20.59% in fat grafting with cultured ASC inclusion in patients with Parry-Romberg disease[57]. Improved retention with ASC or SVF supplementation in facial fat grafting in patients with hemifacial atrophy, facial scarring, and in conjunction with facial cosmetic procedures, has subsequently also been shown by several groups[58-63]. Another comparative study conducted by Gentile et al. compared enriched vs. non-enriched fat grafting in 100 patients with facial scarring and soft tissue deformity, finding improved patient satisfaction in patients treated with enriched fat grafts[64]. A summary of clinical studies is included in Table 1.
Summary of clinical studies including cell-supplemented autologous fat grafting
Title | Authors | Year | Study design | Number of patients | Results |
Progenitor-enriched adipose tissue transplantation as rescue for breast implant complications | Yoshimura et al.[49] | 2010 | Prospective | 15 | No major complications or abnormalities on 12-month imaging. Surviving fat volume at 12 months was 155 ± 50 cc (right) and 143 ± 80 cc (left) following grafting an initial mean of 264 cc |
Cell-assisted lipotransfer for cosmetic breast augmentation: supportive use of adipose-derived stem/stromal cells | Yoshimura et al.[50] | 2008 | Prospective | 40 | Retained augmentation of 100 to 200 mL after a mean fat amount of 270 cc injected |
Autologous cell-enriched fat grafting for breast augmentation | Kamakura et al.[51] | 2011 | Prospective | 20 | Increased circumferential breast measurement of 3.3 cm at 9 months with 75% patient satisfaction at that time |
Prospective trial of adipose-derived regenerative cell (ADRC)-enriched fat grafting for partial mastectomy defects: the RESTORE-2 trial | Perez-Cano et al.[52] | 2012 | Prospective | 67 | No major complications. Fifty patients reported satisfaction with treatment at 12 months; investigators reported satisfaction with 57 patients. Blinded radiographic assessment showed improved breast contour in 54 patients |
A prospective study of the efficacy of cell-assisted lipotransfer with stromal vascular fraction to correct contour deformities of the autologous reconstructed breast | Jeon et al.[54] | 2020 | Prospective | 20 | Higher fat retention in SVF enriched fat grafts at 6 and 12 months |
Ex vivo-expanded autologous adipose tissue-derived stromal cells ensure enhanced fat graft retention in breast augmentation: a randomized controlled clinical trial | Kolle et al.[55] | 2020 | Prospective | 16 | Increased breast volume, retention, and enlargement at 4 months in patients treated with expanded-ASC enriched fat grafting |
Cell-assisted lipotransfer for facial lipoatrophy: efficacy of clinical use of adipose-derived stem cells | Yoshimura et al.[56] | 2008 | Prospective | 6 | Patients with SVF augmented fat transfer had improved clinical outcome scores compared to patients without enriched fat |
Clinical application of human adipose tissue-derived mesenchymal stem cells in progressive hemifacial atrophy (Parry-Romberg disease) with microfat grafting techniques using 3-dimensional computed tomography and 3-dimensional camera | Koh et al.[57] | 2012 | Prospective | 10 | Patients receiving ASC enriched fat grafts showed decreased resorption at 15 months |
Adipose-derived stromal vascular fraction cells and platelet-rich plasma: basic and clinical evaluation for cell-based therapies in patients with scars on the face | Gentile et al.[58] | 2014 | Prospective | 20 | Improved retention of contour at 1 year with SVF-enhanced fat grafting to facial scars |
Quantitative volumetric analysis of progressive hemifacial atrophy corrected using stromal vascular fraction-supplemented autologous fat grafts | Chang et al.[59] | 2013 | Prospective | 20 | Improved patient satisfaction and fat survival seen with SVF augmented fat |
The safety and efficacy of cell-assisted fat grafting to traditional fat grafting in the anterior mid-face: an indirect assessment by 3D imaging | Sasaki[60] | 2015 | Prospective | 236 | SVF fat supplementation to the midface led to increased fat retention at 12 months |
Fat grafts supplemented with adipose-derived stromal cells in the rehabilitation of patients with craniofacial microsomia | Tanikawa et al.[61] | 2013 | Prospective | 14 | Fat supplementation with SVF led to increased fat retention at 6 months compared to control |
Mechanical supplementation with the stromal vascular fraction yields improved volume retention in facial lipotransfer: a 1-year comparative study | Gontijo-de-Amorim et al.[62] | 2017 | Prospective | 30 | SVF fat enrichment showed increased retention via CT imaging at 12 months postoperatively after facial grafting |
Stromal vascular fraction improves the durability of autologous fat temple augmentation - a split-face randomized study using ultrasound biomicroscopy | Roshdy et al.[63] | 2022 | Prospective | 15 | SVF fat enrichment increased fat volume retention at 6 months in bilateral temporal fat grafting |
Adipose tissue-derived stem cells: in vitro and in vivo analysis of a standard and three commercially available cell-assisted lipotransfer techniques | Domenis et al.[40] | 2015 | Prospective | 36 | SVF-enriched fat grafts demonstrated superior retention of thickness at six and 12 months |
Breast reconstruction with enhanced stromal vascular fraction fat grafting: what is the best method? | Gentile et al.[41] | 2015 | Prospective | 50 | Celution and fastem had the highest |
Does stromal vascular fraction ensure a higher survival in autologous fat grafting for breast augmentation? a volumetric study using 3-dimensional laser scanning | Chiu[72] | 2019 | Prospective | 206 | Assessment of volume retention by 3-dimensional laser scanning showed no difference between standard and SVF-supplemented fat grafting |
Is the resorption of grafted fat reduced in cell-assisted lipotransfer for breast augmentation? | Wang et al.[73] | 2015 | Case series | 12 | Patients undergoing CAL had 51.84% fat resorption at 6 months as measured by MRI |
Stem cell enrichment does not warrant a higher graft survival in lipofilling of the breast: a prospective comparative study | Peltoniemi et al.[74] | 2013 | Prospective | 18 | There was no difference in adipose survival or volume retention at six months between patients undergoing traditional fat transfer vs. patients undergoing SVF-supplemented fat transfer |
Lipofilling enriched with adipose-derived mesenchymal stem cells improves soft tissue deformities and reduces scar pigmentation: clinical and instrumental evaluation in plastic surgery | Gentile[64] | 2023 | Prospective | 100 | All patients saw improvement in volume contour and pigmentation, though patients with enriched fat-grafting showed significantly increased satisfaction |
With the role of supplemental cells well-established within CAL, the unique properties of individual subpopulations of ASCs have been explored in the basic science literature[65]. Using flow cytometry gating to remove endothelial and hemopoietic cells from the SVF (CD31-/CD235a-/CD45-), CD34+ cells were isolated from the subset and defined as ASCs. BMPR1a+ isolates had pro-adipogenic potential[66-69]. Within this CD34+ group, individual isolates were further identified with varying properties. Notably, CD146+ cells were found to further augment angiogenesis via increased expression of VEGF, while CD74+ cells exhibited antifibrogenic properties by decreasing TGF-β1 and subsequent collagen production. Although both in vivo and in vitro data have been promising, many logistical barriers remain in attempting clinical implementation of these results, the most salient of which involves multiple hours of laboratory isolation in addition to flow cytometry sorting to provide enough cells for minimal volumes of cell-assisted lipofilling.
The use of CAL has also been studied in irradiated tissues. Radiation is a common adjunct to cancer treatment and can result in skin breakdown, wound healing complications, and poor scarring, among other complications. One of the earliest studies was conducted by Rigotti et al. in 2007, examining 20 patients who underwent chest wall or breast radiotherapy and had clinically irreversible complications including fibrosis, skin ulceration, and skin retraction[70]. Injection of SVF promoted the regeneration of revascularized radiated tissue, and led to a subjective improvement in symptoms. In 2016, Luan et al. used SVF enrichment to transplant fat beneath the irradiated scalp of immunocompromised mice, and found not only increased graft retention but also amelioration of radiation induced skin changes such as improved skin vascularity, dermal thickness, and collagen content[71]. Randomized control trials examining the effects of CAL in irradiated human tissue have yet to be conducted.
CONTROVERSIES AND OVERSIGHT
The evidence regarding the efficacy of using fat grafting and CAL in a variety of clinical contexts is well supported and the body of evidence continues to grow. However, there are certainly studies which show equivocal or non-significant effects of CAL. A prospective study by Chiu et al. in 2019 found no differences in fat retention measured by 3D volumetric scanning between 105 patients undergoing traditional fat grafting and 101 patients undergoing grafting with SVF-enriched fat[72]. Other smaller clinical studies have also supported this claim[73,74]. A meta-analysis conducted by Laloze et al. found CAL to increase retention only in small volume fat grafting of < 100 cc, but also found CAL to increase complication rates without changing the number of subsequent procedures needed compared to patients undergoing traditional fat transfer[75]. The authors do not further elucidate the differences in complication profile and acknowledge the difficulty of meta-analysis in this field given the significant variation in techniques, subjective nature of satisfaction, and limited data examining quantitative volume retention outcomes.
Additionally, there has long been controversy in the procedure relating to its oncologic safety. In 1987, the American Society of Plastic Surgeons prohibited fat grafting to the breasts due to concern that it would impede cancer surveillance, a ban that lasted until 2009[76,77]. Since then, fat grafting has been shown by many to be safe in the setting of prior breast cancer[78-81]. Specifically in the context of CAL, there is a theoretical risk in transplanting multipotent stem cells into a potentially tumorigenic environment where these cells may propagate malignancy or stimulate recurrence. A plethora of basic science work demonstrates increased recurrence, migration, and metastatic potential of breast cancer cells when cultured in isolation with ASCs, which creates significant hesitancy when applied in clinical environments[82-87]. However, clinically-based studies fail to demonstrate any increased potential of malignancy with CAL, a contrast made apparent in a review by Charvet et al.[88]. In one of the most thorough studies on the subject, Calabrese et al. prospectively followed 169 women who either received fat grafting, fat grafting with SVF, or no fat grafting following two-stage breast reconstruction, and found that inclusion of SVF had no significant impact on cancer recurrence[89]. Another study conducted by Mazur et al. compared 3-year recurrence in 56 patients undergoing ASC-supplemented fat grafting with 252 matched patients who did not undergo breast reconstruction and found no difference between the two groups[90]. A recent systematic review by Al Qurashi et al. found an oncologic recurrence incidence of 29/583 in non-irradiated mastectomy patients and 41/517 in breast conservation patients, suggesting overall safety of fat grafting after oncologic surgery[91]. As the use of ASCs and CAL broadens, more refined data are likely to emerge.
Beyond questions of oncologic safety, there has also been codification by the United States Food and Drug Administration (FDA) acknowledging the use of adipose-derived stem cell isolation and supplementation as beyond “minimally manipulated” and therefore requiring specific pre-market approval, based on a ruling by the Southern District of Florida in 2019[57,92,93]. The report cites the processing of structural tissue and its transformation into a product that “alter(s) the original relevant characteristics of the tissue relating to the tissue’s utility for reconstruction, repair, or replacement” as beyond the scope of surgical reconstruction. The only currently FDA-approved use of stem cells relates to hematopoietic progenitor cells, thus making regular clinical use of CAL a legal boundary for most providers. The European Medicines Agency has come up with similar guidelines and restrictions for the use of ADSCs in the European Union[94].
FUTURE DIRECTIONS
As clinical use of CAL continues to increase, more rigorous, large cohort studies are needed to both validate safety and ensure no increased risk of tumorigenesis. Further studies will need to answer questions regarding the optimal timing of CAL in relation to adjuvant chemotherapy or radiation, as well as ascertain the expected volumetric clinical survival in these patients. The ratio of stromal vascular fraction to fat in the clinical setting needs to be further elucidated, as well as if there is a dose-dependent response as seen in laboratory models. Additionally, isolation of ADC sub-populations and their implementation may be a potential tool to optimize this technique, although significant logistical barriers remain prevalent. Just as there has been the development of multiple systems or devices to streamline standard fat grafting, we anticipate an increase in products to streamline the isolation of progenitor cells from lipoaspirate so as to keep the cell supplementation within the same surgical procedure and obviate the need for additional manipulation, culture-expansion, or procedures for CAL.
CONCLUSION
Adipose-derived therapies, such as CAL and engineered tissues, are a burgeoning field that shows promise in both cosmetic and reconstructive practice. To date, there have been several, mostly retrospective, studies regarding fat harvest, SVF isolation, and indications for the use of CAL. However, large cohort prospective trials remain to be done. In addition to currently known uses, the concept of cryopreserved SVF has been an area of increased study in mouse models[95-97] in an attempt to alleviate the logistical burdens SVF isolation requires, which in turn may also provide an avenue for ASC subpopulation use in the future. With future studies and further refinements in technique, engineered adipose therapeutics have the potential to increase the consistency of survival of grafted fat and thus become a standard approach in the reconstructive armamentarium.
DECLARATIONS
Authors’ contributions
Made substantial contributions to the conception and writing of the review: Ghosh K, Patel RA, Hanson SE
Availability of data and materials
Not applicable.
Financial support and sponsorship
Dr. Hanson has received funding from the Plastic Surgery Foundation (PSF-MTF Biologics grant) and NIH (R21 EB035242).
Conflicts of interest
Hanson SE is the Guest Editor of the Special Issue of “New Perspectives in Fat Grafting.” Hanson SE has previously consulted for Sientra, Inc. The other author has declared that there are no conflicts of interest.
Ethical approval and consent to participate
Not applicable.
Consent for publication
Not applicable.
Copyright
© The Author(s) 2024.
REFERENCES
1. Neuber F. Fettransplantation. Available from: https://www.scirp.org/reference/referencespapers?referenceid=1812808 [Last accessed on 27 Sep 2024].
2. Bellini E, Grieco MP, Raposio E. The science behind autologous fat grafting. Ann Med Surg (Lond) 2017;24:65-73.
3. Bircoll M. Cosmetic breast augmentation utilizing autologous fat and liposuction techniques. Plast Reconstr Surg 1987;79:267-71.
4. Cortese A, Savastano G, Felicetta L. Free fat transplantation for facial tissue augmentation. J Oral Maxillofac Surg 2000;58:164-9.
6. Simonacci F, Bertozzi N, Grieco MP, Grignaffini E, Raposio E. Procedure, applications, and outcomes of autologous fat grafting. Ann Med Surg (Lond) 2017;20:49-60.
7. Sinno S, Wilson S, Brownstone N, Levine SM. Current thoughts on fat grafting: using the evidence to determine fact or fiction. Plast Reconstr Surg 2016;137:818-24.
8. Boschert MT, Beckert BW, Puckett CL, Concannon MJ. Analysis of lipocyte viability after liposuction. Plast Reconstr Surg 2002;109:761-5.
9. Crawford JL, Hubbard BA, Colbert SH, Puckett CL. Fine tuning lipoaspirate viability for fat grafting. Plast Reconstr Surg 2010;126:1342-8.
10. Billings E Jr, May JW Jr. Historical review and present status of free fat graft autotransplantation in plastic and reconstructive surgery. Plast Reconstr Surg 1989;83:368-81.
11. Doi K, Ogata F, Eto H, et al. Differential contributions of graft-derived and host-derived cells in tissue regeneration/remodeling after fat grafting. Plast Reconstr Surg 2015;135:1607-17.
12. Kato H, Mineda K, Eto H, et al. Degeneration, regeneration, and cicatrization after fat grafting: dynamic total tissue remodeling during the first 3 months. Plast Reconstr Surg 2014;133:303e-13e.
13. Eto H, Suga H, Matsumoto D, et al. Characterization of structure and cellular components of aspirated and excised adipose tissue. Plast Reconstr Surg 2009;124:1087-97.
14. Rodbell M. Metabolism of isolated fat cells: II. The similar effects of phospholipase C (clostridium perfringens alpha toxin) and of insulin on glucose and amino acid metabolism. J Biol Chem ;241:130-9.
15. Bunnell BA, Flaat M, Gagliardi C, Patel B, Ripoll C. Adipose-derived stem cells: isolation, expansion and differentiation. Methods 2008;45:115-20.
16. Fu S, Luan J, Xin M, Wang Q, Xiao R, Gao Y. Fate of adipose-derived stromal vascular fraction cells after co-implantation with fat grafts: evidence of cell survival and differentiation in ischemic adipose tissue. Plast Reconstr Surg 2013;132:363-73.
17. Zuk PA, Zhu M, Mizuno H, et al. Multilineage cells from human adipose tissue: implications for cell-based therapies. Tissue Eng 2001;7:211-28.
18. Strioga M, Viswanathan S, Darinskas A, Slaby O, Michalek J. Same or not the same? Stem Cells Dev 2012;21:2724-52.
19. Suga H, Eto H, Aoi N, et al. Adipose tissue remodeling under ischemia: death of adipocytes and activation of stem/progenitor cells. Plast Reconstr Surg 2010;126:1911-23.
20. Hong KY, Yim S, Kim HJ, et al. The fate of the adipose-derived stromal cells during angiogenesis and adipogenesis after cell-assisted lipotransfer. Plast Reconstr Surg 2018;141:365-75.
21. Puissant B, Barreau C, Bourin P, et al. Immunomodulatory effect of human adipose tissue-derived adult stem cells: comparison with bone marrow mesenchymal stem cells. Br J Haematol 2005;129:118-29.
22. Garza RM, Rennert RC, Paik KJ, et al. Studies in fat grafting: part IV. Adipose-derived stromal cell gene expression in cell-assisted lipotransfer. Plast Reconstr Surg 2015;135:1045-55.
23. Suh A, Pham A, Cress MJ, et al. Adipose-derived cellular and cell-derived regenerative therapies in dermatology and aesthetic rejuvenation. Ageing Res Rev 2019;54:100933.
24. Borrelli MR, Patel RA, Sokol J, et al. Fat chance: the rejuvenation of irradiated skin. Plast Reconstr Surg Glob Open 2019;7:e2092.
25. Coleman SR. Long-term survival of fat transplants: controlled demonstrations. Aesthetic Plast Surg 1995;19:421-5.
27. Tabit CJ, Slack GC, Fan K, Wan DC, Bradley JP. Fat grafting versus adipose-derived stem cell therapy: distinguishing indications, techniques, and outcomes. Aesthetic Plast Surg 2012;36:704-13.
28. Harats M, Millet E, Jaeger M, et al. Adipocytes viability after suction-assisted lipoplasty: does the technique matter? Aesthetic Plast Surg 2016;40:578-83.
29. Hanson SE, Garvey PB, Chang EI, et al. A prospective, randomized comparison of clinical outcomes with different processing techniques in autologous fat grafting. Plast Reconstr Surg 2022;150:955-62.
30. Keck M, Kober J, Riedl O, et al. Power assisted liposuction to obtain adipose-derived stem cells: impact on viability and differentiation to adipocytes in comparison to manual aspiration. J Plast Reconstr Aesthet Surg 2014;67:e1-8.
31. Duscher D, Atashroo D, Maan ZN, et al. Ultrasound-assisted liposuction does not compromise the regenerative potential of adipose-derived stem cells. Stem Cells Transl Med 2016;5:248-57.
32. Trivisonno A, Di Rocco G, Cannistra C, et al. Harvest of superficial layers of fat with a microcannula and isolation of adipose tissue-derived stromal and vascular cells. Aesthet Surg J 2014;34:601-13.
33. Alharbi Z, Opländer C, Almakadi S, Fritz A, Vogt M, Pallua N. Conventional vs. micro-fat harvesting: how fat harvesting technique affects tissue-engineering approaches using adipose tissue-derived stem/stromal cells. J Plast Reconstr Aesthet Surg 2013;66:1271-8.
34. Chen YW, Wang JR, Liao X, et al. Effect of suction pressures on cell yield and functionality of the adipose-derived stromal vascular fraction. J Plast Reconstr Aesthet Surg 2017;70:257-66.
35. Sterodimas A, de Faria J, Nicaretta B, Papadopoulos O, Papalambros E, Illouz YG. Cell-assisted lipotransfer. Aesthet Surg J 2010;30:78-81.
36. Aronowitz JA, Ellenhorn JDI. Adipose stromal vascular fraction isolation: a head-to-head comparison of four commercial cell separation systems. Plast Reconstr Surg 2013;132:932e-9e.
37. Chang H, Do BR, Che JH, et al. Safety of adipose-derived stem cells and collagenase in fat tissue preparation. Aesthetic Plast Surg 2013;37:802-8.
38. Condé-Green A, Kotamarti VS, Sherman LS, et al. Shift toward mechanical isolation of adipose-derived stromal vascular fraction: review of upcoming techniques. Plast Reconstr Surg Glob Open 2016;4:e1017.
39. Tiryaki T, Condé-Green A, Cohen SR, Canikyan S, Kocak P. A 3-step mechanical digestion method to harvest adipose-derived stromal vascular fraction. Plast Reconstr Surg Glob Open 2020;8:e2652.
40. Domenis R, Lazzaro L, Calabrese S, et al. Adipose tissue derived stem cells: in vitro and in vivo analysis of a standard and three commercially available cell-assisted lipotransfer techniques. Stem Cell Res Ther 2015;6:2.
41. Gentile P, Scioli MG, Orlandi A, Cervelli V. Breast reconstruction with enhanced stromal vascular fraction fat grafting: what is the best method? Plast Reconstr Surg Glob Open 2015;3:e406.
42. Gentile P, Cervelli V, De Fazio D, Calabrese C, Scioli MG, Orlandi A. Mechanical and enzymatic digestion of autologous fat grafting (A-FG): fat volume maintenance and ad-svfs amount in comparison. Aesthetic Plast Surg 2023;47:2051-62.
43. Di Taranto G, Cicione C, Visconti G, et al. Qualitative and quantitative differences of adipose-derived stromal cells from superficial and deep subcutaneous lipoaspirates: a matter of fat. Cytotherapy 2015;17:1076-89.
44. Faustini M, Bucco M, Chlapanidas T, et al. Nonexpanded mesenchymal stem cells for regenerative medicine: yield in stromal vascular fraction from adipose tissues. Tissue Eng Part C Methods 2010;16:1515-21.
45. Tsekouras A, Mantas D, Tsilimigras DI, Moris D, Kontos M, Zografos GC. Comparison of the viability and yield of adipose-derived stem cells (ASCs) from different donor areas. In Vivo 2017;31:1229-34.
46. Grasys J, Kim BS, Pallua N. Content of soluble factors and characteristics of stromal vascular fraction cells in lipoaspirates from different subcutaneous adipose tissue depots. Aesthet Surg J 2016;36:831-41.
47. Iyyanki T, Hubenak J, Liu J, Chang EI, Beahm EK, Zhang Q. Harvesting technique affects adipose-derived stem cell yield. Aesthet Surg J 2015;35:467-76.
48. Matsumoto D, Sato K, Gonda K, et al. Cell-assisted lipotransfer: supportive use of human adipose-derived cells for soft tissue augmentation with lipoinjection. Tissue Eng 2006;12:3375-82.
49. Yoshimura K, Asano Y, Aoi N, et al. Progenitor-enriched adipose tissue transplantation as rescue for breast implant complications. Breast J 2010;16:169-75.
50. Yoshimura K, Sato K, Aoi N, Kurita M, Hirohi T, Harii K. Cell-assisted lipotransfer for cosmetic breast augmentation: supportive use of adipose-derived stem/stromal cells. Aesthetic Plast Surg 2008;32:48-55.
51. Kamakura T, Ito K. Autologous cell-enriched fat grafting for breast augmentation. Aesthetic Plast Surg 2011;35:1022-30.
52. Pérez-Cano R, Vranckx JJ, Lasso JM, et al. Prospective trial of adipose-derived regenerative cell (ADRC)-enriched fat grafting for partial mastectomy defects: the RESTORE-2 trial. Eur J Surg Oncol 2012;38:382-9.
53. Li M, Chen C. The efficacy of cell-assisted lipotransfer versus conventional lipotransfer in breast augmentation: a systematic review and meta-analysis. Aesthetic Plast Surg 2021;45:1478-86.
54. Jeon HJ, Choi DH, Lee JH, et al. A prospective study of the efficacy of cell-assisted lipotransfer with stromal vascular fraction to correct contour deformities of the autologous reconstructed breast. Aesthetic Plast Surg 2021;45:853-63.
55. Kølle ST, Duscher D, Taudorf M, et al. Ex vivo-expanded autologous adipose tissue-derived stromal cells ensure enhanced fat graft retention in breast augmentation: a randomized controlled clinical trial. Stem Cells Transl Med 2020;9:1277-86.
56. Yoshimura K, Sato K, Aoi N, et al. Cell-assisted lipotransfer for facial lipoatrophy: efficacy of clinical use of adipose-derived stem cells. Dermatol Surg 2008;34:1178-85.
57. Koh KS, Oh TS, Kim H, et al. Clinical application of human adipose tissue-derived mesenchymal stem cells in progressive hemifacial atrophy (Parry-Romberg disease) with microfat grafting techniques using 3-dimensional computed tomography and 3-dimensional camera. Ann Plast Surg 2012;69:331-7.
58. Gentile P, De Angelis B, Pasin M, et al. Adipose-derived stromal vascular fraction cells and platelet-rich plasma: basic and clinical evaluation for cell-based therapies in patients with scars on the face. J Craniofac Surg 2014;25:267-72.
59. Chang Q, Li J, Dong Z, Liu L, Lu F. Quantitative volumetric analysis of progressive hemifacial atrophy corrected using stromal vascular fraction-supplemented autologous fat grafts. Dermatol Surg 2013;39:1465-73.
60. Sasaki GH. The safety and efficacy of cell-assisted fat grafting to traditional fat grafting in the anterior mid-face: an indirect assessment by 3D imaging. Aesthetic Plast Surg 2015;39:833-46.
61. Tanikawa DYS, Aguena M, Bueno DF, Passos-Bueno MR, Alonso N. Fat grafts supplemented with adipose-derived stromal cells in the rehabilitation of patients with craniofacial microsomia. Plast Reconstr Surg 2013;132:141-52.
62. Gontijo-de-Amorim NF, Charles-de-Sá L, Rigotti G. Mechanical supplementation with the stromal vascular fraction yields improved volume retention in facial lipotransfer: a 1-year comparative study. Aesthet Surg J 2017;37:975-85.
63. Roshdy OH, Abdallah WI, Farid CI, Mehanna RA, Bayoumi NH, Ismail AI. Stromal vascular fraction improves the durability of autologous fat temple augmentation - a split-face randomized study using ultrasound biomicroscopy. J Plast Reconstr Aesthet Surg 2022;75:1870-7.
64. Gentile P. Lipofilling enriched with adipose-derived mesenchymal stem cells improves soft tissue deformities and reduces scar pigmentation: clinical and instrumental evaluation in plastic surgery. Aesthetic Plast Surg 2023;47:2063-73.
65. Li H, Zimmerlin L, Marra KG, Donnenberg VS, Donnenberg AD, Rubin JP. Adipogenic potential of adipose stem cell subpopulations. Plast Reconstr Surg 2011;128:663-72.
66. Borrelli MR, Patel RA, Blackshear C, et al. CD34+CD146+ adipose-derived stromal cells enhance engraftment of transplanted fat. Stem Cells Transl Med 2020;9:1389-400.
67. Borrelli MR, Patel RA, Adem S, et al. The antifibrotic adipose-derived stromal cell: grafted fat enriched with CD74+ adipose-derived stromal cells reduces chronic radiation-induced skin fibrosis. Stem Cells Transl Med 2020;9:1401-13.
68. Bourin P, Bunnell BA, Casteilla L, et al. Stromal cells from the adipose tissue-derived stromal vascular fraction and culture expanded adipose tissue-derived stromal/stem cells: a joint statement of the International Federation for Adipose Therapeutics and Science (IFATS) and the International Society for Cellular Therapy (ISCT). Cytotherapy 2013;15:641-8.
69. Zielins ER, Paik K, Ransom RC, et al. Enrichment of adipose-derived stromal cells for bmpr1a facilitates enhanced adipogenesis. Tissue Eng Part A 2016;22:214-21.
70. Rigotti G, Marchi A, Galiè M, et al. Clinical treatment of radiotherapy tissue damage by lipoaspirate transplant: a healing process mediated by adipose-derived adult stem cells. Plast Reconstr Surg 2007;119:1409-22.
71. Luan A, Duscher D, Whittam AJ, et al. Cell-assisted lipotransfer improves volume retention in irradiated recipient sites and rescues radiation-induced skin changes. Stem Cells 2016;34:668-73.
72. Chiu CH. Does stromal vascular fraction ensure a higher survival in autologous fat grafting for breast augmentation? Aesthet Surg J 2019;39:41-52.
73. Wang L, Luo X, Lu Y, Fan ZH, Hu X. Is the resorption of grafted fat reduced in cell-assisted lipotransfer for breast augmentation? Ann Plast Surg 2015;75:128-34.
74. Peltoniemi HH, Salmi A, Miettinen S, et al. Stem cell enrichment does not warrant a higher graft survival in lipofilling of the breast: a prospective comparative study. J Plast Reconstr Aesthet Surg 2013;66:1494-503.
75. Laloze J, Varin A, Gilhodes J, et al. Cell-assisted lipotransfer: friend or foe in fat grafting? J Tissue Eng Regen Med 2018;12:e1237-50.
76. Report on autologous fat transplantation. ASPRS ad-hoc committee on new procedures, September 30, 1987. Plast Surg Nurs 1987;7:140-1.
77. Surgeons ASoP. Fat transfer/fat graft and fat injection: ASPS guiding principles. Available from: https://www.plasticsurgery.org/Documents/Health-Policy/Principles/principle-2015-post-mastectomy-fat-grafting.pdf [Last accessed on 28 Sep 2024].
78. Tukiama R, Vieira RAC, Facina G, da Cunha Leal P, Zucca-Matthes G. Oncologic safety of autologous fat grafting after breast cancer surgical treatment: a matched cohort study. Plast Reconstr Surg 2021;148:11-20.
79. Cohen O, Lam G, Karp N, Choi M. Determining the oncologic safety of autologous fat grafting as a reconstructive modality: an institutional review of breast cancer recurrence rates and surgical outcomes. Plast Reconstr Surg 2017;140:382e-92e.
80. Hanson SE, Kapur SK, Garvey PB, et al. Oncologic safety and surveillance of autologous fat grafting following breast conservation therapy. Plast Reconstr Surg 2020;146:215-25.
81. Mestak O, Hromadkova V, Fajfrova M, Molitor M, Mestak J. Evaluation of oncological safety of fat grafting after breast-conserving therapy: a prospective study. Ann Surg Oncol 2016;23:776-81.
82. Goto H, Shimono Y, Funakoshi Y, et al. Adipose-derived stem cells enhance human breast cancer growth and cancer stem cell-like properties through adipsin. Oncogene 2019;38:767-79.
83. Eterno V, Zambelli A, Pavesi L, et al. Adipose-derived Mesenchymal Stem Cells (ASCs) may favour breast cancer recurrence via HGF/c-Met signaling. Oncotarget 2014;5:613-33.
84. Gehmert S, Gehmert S, Prantl L, Vykoukal J, Alt E, Song YH. Breast cancer cells attract the migration of adipose tissue-derived stem cells via the PDGF-BB/PDGFR-beta signaling pathway. Biochem Biophys Res Commun 2010;398:601-5.
85. Kuhbier JW, Bucan V, Reimers K, et al. Observed changes in the morphology and phenotype of breast cancer cells in direct co-culture with adipose-derived stem cells. Plast Reconstr Surg 2014;134:414-23.
86. Rowan BG, Gimble JM, Sheng M, et al. Human adipose tissue-derived stromal/stem cells promote migration and early metastasis of triple negative breast cancer xenografts. PLoS One 2014;9:e89595.
87. Orecchioni S, Gregato G, Martin-Padura I, et al. Complementary populations of human adipose CD34+ progenitor cells promote growth, angiogenesis, and metastasis of breast cancer. Cancer Res 2013;73:5880-91.
88. Charvet HJ, Orbay H, Wong MS, Sahar DE. The oncologic safety of breast fat grafting and contradictions between basic science and clinical studies: a systematic review of the recent literature. Ann Plast Surg 2015;75:471-9.
89. Calabrese C, Kothari A, Badylak S, et al. Oncological safety of stromal vascular fraction enriched fat grafting in two-stage breast reconstruction after nipple sparing mastectomy: long-term results of a prospective study. Eur Rev Med Pharmacol Sci 2018;22:4768-77.
90. Mazur S, Zołocińska A, Siennicka K, Janik-Kosacka K, Chrapusta A, Pojda Z. Safety of adipose-derived cell (stromal vascular fraction - SVF) augmentation for surgical breast reconstruction in cancer patients. Adv Clin Exp Med 2018;27:1085-90.
91. Al Qurashi AA, Shah Mardan QNM, Alzahrani IA, et al. Efficacy of exclusive fat grafting for breast reconstruction: an updated systematic review and meta-analysis. Aesthetic Plast Surg 2024; doi: 10.1007/s00266-024-03978-3.
92. Administration USFaD. Federal court issues decision holding that US Stem Cell clinics and owner adulterated and misbranded stem cell products in violation of the law. Available from: https://www.fda.gov/news-events/press-announcements/federal-court-issues-decision-holding-us-stem-cell-clinics-and-owner-adulterated-and-misbranded-stem [Last accessed on 28 Sep 2024].
93. Services USDoHaH. Regulatory considerations for human cells, tissues, and cellular andtissue-based products: minimal manipulation and homologous use. Available from: https://www.fda.gov/regulatory-information/search-fda-guidance-documents/regulatory-considerations-human-cells-tissues-and-cellular-and-tissue-based-products-minimal [Last accessed on 28 Sep 2024].
94. Raposio E, Ciliberti R. Clinical use of adipose-derived stem cells: European legislative issues. Ann Med Surg (Lond) 2017;24:61-4.
95. Bae YC, Song JS, Bae SH, Kim JH. Effects of human adipose-derived stem cells and stromal vascular fraction on cryopreserved fat transfer. Dermatol Surg 2015;41:605-14.
96. Zanata F, Bowles A, Frazier T, et al. Effect of cryopreservation on human adipose tissue and isolated stromal vascular fraction cells: in vitro and in vivo analyses. Plast Reconstr Surg 2018;141:232e-43e.
Cite This Article

How to Cite
Ghosh, K.; Patel, R. A.; Hanson, S. E. Cell-supplemented autologous fat grafting: a review from bench to bedside. Plast. Aesthet. Res. 2024, 11, 50. http://dx.doi.org/10.20517/2347-9264.2024.70
Download Citation
Export Citation File:
Type of Import
Tips on Downloading Citation
Citation Manager File Format
Type of Import
Direct Import: When the Direct Import option is selected (the default state), a dialogue box will give you the option to Save or Open the downloaded citation data. Choosing Open will either launch your citation manager or give you a choice of applications with which to use the metadata. The Save option saves the file locally for later use.
Indirect Import: When the Indirect Import option is selected, the metadata is displayed and may be copied and pasted as needed.
About This Article
Special Issue
Copyright
Data & Comments
Data
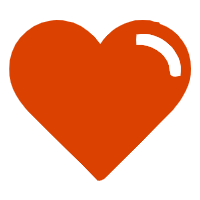
Comments
Comments must be written in English. Spam, offensive content, impersonation, and private information will not be permitted. If any comment is reported and identified as inappropriate content by OAE staff, the comment will be removed without notice. If you have any queries or need any help, please contact us at support@oaepublish.com.