Robotic-assisted microsurgery using the MUSA-robot: evaluation of the learning curve in three clinical pilot trials
Abstract
Aim: Current microsurgical procedures are limited by the physiological tremor and dexterity of the surgeon. The MicroSurgical Assistant (MUSA, Microsure), the world’s first robotic platform for (super)microsurgery can aid in resolving issues encountered during microsurgery. This study presents an overview of the operating times and Structured Assessment of Microsurgery Skills (SAMS) scores to assess the duration and quality of microsurgical anastomoses for three microsurgical procedures currently performed using the MUSA.
Methods: This study integrates data from one ongoing randomized controlled trial focusing on robotic-assisted lymphaticovenous anastomosis, along with findings from two separate prospective pilot studies concerning digital nerve repair and free tissue transplantation. SAMS scores and time needed per anastomosis were used to evaluate the quality and learning curve of the MUSA-assisted procedures.
Results: Thirty-five robotic-assisted procedures were analyzed, including 18 lymphaticovenous anastomoses, 9 digital nerve repairs, and 8 free tissue transplantations. All procedures showed a trend of a decrease in the time needed to perform the procedure. Moreover, the mean overall SAMS scores for all three procedures were rated above ‘satisfactory’, with all procedures demonstrating a consistent trend of increasing SAMS scores over time.
Conclusion: The evaluation of anastomosis’ quality in the initial cohorts of patients undergoing robotic-assisted microsurgery using MUSA indicates satisfying outcomes across all three types of procedures. The reduction in anastomosis time and the improvement in SAMS scores imply an ongoing learning process among the operating surgeons. Subsequent reports are expected to provide information on reaching a plateau phase in procedural efficiency.
Keywords
INTRODUCTION
Supermicrosurgery necessitates exceptional hand-eye coordination, precise tissue handling, dexterity, and a smooth operative flow[1]. These attributes are susceptible to various internal and external surgeon-related limitations, including the surgeon’s abilities, tremors, fatigue, and the effects of aging. Additionally, engagement in supermicrosurgery can adversely affect the surgeon due to prolonged static positions, suboptimal ergonomic postures, and overall muscular fatigue[2]. To address these challenges, the logical progression in advancing supermicrosurgery involves the implementation of robotic assistance[3].
Robotic-assisted microsurgery holds promise in refining supermicrosurgical procedures. This is achieved by offering ergonomic designs and improved instrument handling. Consequently, robotic-assisted microsurgery can provide physical relief for surgeons, improve and standardize the quality of therapy, ultimately resulting in enhanced patient outcomes[4].
A collaborative effort between Maastricht University Medical Center (MUMC+) and Eindhoven University of Technology (TUE) has led to the development of the world’s robotic platform specifically designed for (super)microsurgery, known as the MicroSurgical Assistant (MUSA) (MicroSure, Eindhoven, the Netherlands). MUSA is designed to surmount human limitations and seamlessly integrate into the existing infrastructure of the operating theatre. Through the implementation of motion scaling and filtration of the surgeon’s physiological tremor, this system optimizes the surgeon’s dexterity and precision during microsurgical procedures.
In both a preclinical study on silicone vessels[5] and a rodent study on the abdominal aorta (1.8 to 2.4 mm) and femoral artery (0.7 to 0.8 mm)[6], the Structured Assessment of Microsurgical Skills (SAMS) score[7] was used to assess the quality of the anastomoses. Additionally, the time to perform an anastomosis was registered. In both studies, manual microsurgery had overall higher SAMS scores compared to robotic-assisted microsurgery. However, a steep learning curve and a decrease in time were reported for indicative skill and overall performance in the robotic-assisted microsurgery group of both studies[5,6].
Subsequently, the first randomized clinical trial comparing the quality of conventional lymphaticovenous anastomosis with a robotic-assisted microsurgery procedure was performed. This trial involved twenty patients with breast cancer-related lymphedema[8]. The results demonstrated comparable clinical outcomes between the robotic-assisted- and manual lymphaticovenous anastomosis, with an improved quality of life observed at the twelve-month follow-up and a reduction in the use of compression garments[8].
The success of incorporating MUSA in the operating theatre resulted in subsequent ongoing trials where MUSA is being used for digital nerve repairs and free tissue transplantation. In this present study, an overview of the SAMS score and operating time will be presented to assess the time and quality of the three microsurgical procedures currently performed with the MUSA (lymphaticovenous anastomosis, digital nerve repair, and free tissue transplantation).
METHODS
Research design
This study combines the data of one ongoing randomized controlled trial on robotic-assisted lymphaticovenous anastomosis and two prospective pilot studies on respectively digital nerve repair and free tissue transplantation.
Setting
The Plastic and Reconstructive Surgery department at MUMC+ possesses extensive expertise in microsurgery. In the Netherlands, this department is among the largest reconstruction centers, conducting an average of 5-6 microsurgical autologous reconstructions per week.
The MUSA-robot
The MUSA is a robotic platform that is specifically designed for microsurgery. It is a compact and lightweight robot and is compatible with existing surgical microscopes and exoscopes. With the use of adapters, it can be paired with any conventional (super)microsurgical instruments. MUSA comprises table-mounted manipulators that can be adjusted in height based on the operative field’s location. The surgeon, with a direct view of the operation site, operates using joystick-like master manipulators [Figure 1]. The device software enables the manipulators to filter out tremors, and motion scaling is facilitated through foot pedals. What sets the MUSA robot apart from other robot platforms is that it is specifically designed for microsurgery and enables easy integration into traditional surgical setups. Its compatibility with current supermicrosurgical instruments enhances its versatility, allowing it to perform both microsurgical and supermicrosurgical procedures. Moreover, by minimizing the need for new disposable materials, the MUSA robot contributes to cost reduction[8].
Microsurgical procedures
Robotic-assisted lymphaticovenous anastomosis
Lymphaticovenular anastomosis (LVA) is carried out under local anesthesia. Photos of preoperative near-infrared imaging provide insights into the location of functional lymphatic vessels, guiding the selection of incision sites. Subsequently, the patient’s limb is prepared for surgery. Before making the incisions, a mixture of bupivacaine and epinephrine (2.5 mg∙mL, 5 mcg∙mL) is injected at the incision sites to achieve both local anesthesia and optimal hemostasis. The surgical procedure is further conducted under a surgical microscope.
Once a viable lymphatic vessel is identified, it is connected to a similarly sized adjacent recipient venule in the subdermal plane with the assistance of the MUSA robot. Typically, an end-to-end anastomosis is preferred if both the lymphatic vessel and venule exhibit comparable calibers; otherwise, an end-to-side approach may be adopted. The anastomosis is created with an 11-0 Ethilon suture, necessitating the use of superfine microsurgical instruments. After the anastomosis is completed, its patency is verified by observing the passage of blood from the venule through the anastomosis into the lymphatic channel. Finally, the superficial wound is closed using 4-0 Ethilon sutures, covered by adhesive plasters. These procedures were exclusively performed by a single microsurgeon with extensive expertise in this field (SSQS).
Robotic-assisted digital nerve repair
Identical to standard treatment, robotic-assisted digital nerve repair (DNR) can be performed under wide-awake local anesthesiology[9]. Prior to the procedure, the operating area is injected with bupivacaine and epinephrine (2.5 mg∙mL, 5 mcg∙mL). The patient’s arm is sterilized, and sterile drapes are applied. Using loupe magnification, the microsurgeon explores the injured site to dissect the injured nerves and other structures. Following debridement of the wound and preparation of the nerve ends, the nerve is repaired using the MUSA system in combination with a surgical microscope. The decision for primary repair of the nerve laceration and the quantity and size of sutures required is determined by the operating microsurgeon. Ethilon 9.0 and 10.0 sutures were used to restore proper and common palmar digital nerves in the hand. Any accompanying vascular or tendon injuries are also addressed and repaired during the same surgical procedure. The robotic-assisted DNR procedures were performed by a single microsurgeon with extensive expertise (TvM).
Robotic-assisted free tissue transplantation
Depending on the indication for the reconstruction of the lower limb, colleagues of other specialties can operate together in one operation (e.g., tumor removal or treatment of fractures). After harvesting a free flap from the patient’s body, the flap is positioned within the defect, and the blood vessels and nerves are reattached to the recipient site. The preparation involves manual preparation aided by loupe magnification. Subsequently, the final connection of the vessels, and if necessary, nerves, is executed using 9.0 or 10.0 micro sutures under microscope magnification, with assistance from the MUSA robotic system. The robotic-assisted free flap procedures were performed by three microsurgeons (RvdH, SSQS, and TvM). The indications for lower extremity reconstruction were acute injuries, fracture nonunions, and melanoma resections. The free flaps used were Antero Lateral Thigh flap, Radial forearm flap, and Superficial Circumflex Iliac artery Perforator flap.
Microsurgery technique assessment
Evaluation of the quality of the performed microsurgical technique in this study utilized the SAMS method, an established and validated tool employed for the objective assessment of microsurgical skills in a clinical setting[7]. To maintain objectivity, all microsurgeries conducted during the study were captured using a digital microscope system. These recorded videos were assessed by one to three independent consultants not affiliated with the research team.
In applying the SAMS method, the assessment of anastomosis technique centered on four critical factors: dexterity, visuospatial ability, operative flow, and judgment. Each of these factors comprised three items, rated on a five-point Likert scale, with higher scores signifying superior anastomosis technique quality[7]. Additionally, the overall performance of the technique underwent evaluation using a five-point Likert scale. This scale ranges from 1 (bad) through 2 (borderline), 3 (satisfactory), and 4 (good) to 5 (excellent).
The duration required for each anastomosis or nerve repair was documented using the recorded procedure videos. Timing commenced with the initial contact of the suture needle with the vessel or nerve and concluded upon the cutting of the final suture, ensuring accurate time measurements.
Methods of data analysis (including statistical analyses)
Descriptive statistics were used in this analysis, along with trendlines for the anastomosis time and SAMS score. Continuous variables were presented as mean [standard deviation (SD)].
RESULTS
A total number of 35 procedures were performed in this analysis. These consisted of 18 robotic-assisted lymphaticovenous anastomoses, 9 robotic-assisted digital nerve repairs, and 8 robotic-assisted free flaps.
Time
The mean time to perform one robotic-assisted lymphaticovenous anastomosis was 16 min, with a range of 5 to 33 min. For the robotic-assisted digital nerve repair, the mean time was 16 min, with a range of 7 to 29 min. For the robotic-assisted free flap, the mean time was 34 min. The range in this group was 21 to 63 min. All procedures showed a trend of a decrease in the time needed to perform the procedure [Figure 2].
SAMS score
The mean overall SAMS scores for all three procedures were rated above three (satisfactory), with robotic-assisted LVA achieving the highest score of 3.67 (SD 0.38), followed by robotic-assisted Free Flap at 3.38 (SD 0.74) and robotic-assisted DNR at 3.22 (SD 0.44) [Table 1]. In terms of dexterity, most scores ranged between three (satisfactory) and four (good), with robotic-assisted DNR and robotic-assisted Free Flap scoring higher for steadiness [5.0 (SD 0.0) and 4.5 (SD 0.93), respectively], and robotic-assisted DNR showing superior tissue handling (4.25 SD 0.67). The mean visuospatial ability scores also fell within the range of three (satisfactory) to four (good), with robotic-assisted DNR scoring higher for suture placement (4.78 SD 0.67). Operative flow scores were above three (satisfactory) for both robotic-assisted LVA and robotic-assisted Free Flap, while robotic-assisted DNR ranged between two (borderline) and three (satisfactory). Irrigation quality was consistently good to excellent across all procedures.
SAMS scores were mostly above satisfactory in all three procedures
SAMS category | Robotic-assisted LVA (n = 18) | Robotic-assisted DNR (n = 9) | Robotic-assisted Free Flap (n = 8) | ||
Overall performance | Mean | 3.67 | 3.22 | 3.38 | |
SD | 0.38 | 0.44 | 0.74 | ||
Dexterity | Steadiness | Mean | 3.41 | 5.00 | 4.50 |
SD | 0.43 | 0.00 | 0.93 | ||
Instrument handling | Mean | 3.12 | 3.33 | 3.63 | |
SD | 0.53 | 1.00 | 0.74 | ||
Tissue handling | Mean | 3.30 | 4.22 | 3.63 | |
SD | 0.49 | 0.67 | 0.74 | ||
Visuospatial ability | Dissection | Mean | 3.43 | 3.88 | 4.00 |
SD | 0.70 | 0.64 | 0.93 | ||
Suture placement | Mean | 3.71 | 4.78 | 4.00 | |
SD | 0.53 | 0.67 | 0.82 | ||
Knot technique | Mean | 3.76 | 3.44 | 4.00 | |
SD | 0.49 | 0.73 | 0.58 | ||
Operative flow | Steps | Mean | 3.26 | 2.56 | 3.00 |
SD | 0.67 | 0.73 | 0.00 | ||
Motion | Mean | 3.06 | 2.67 | 3.29 | |
SD | 0.72 | 0.71 | 0.76 | ||
Speed | Mean | 3.08 | 2.44 | 3.57 | |
SD | 0.75 | 0.73 | 0.53 | ||
Judgement | Irrigation | Mean | 4.18 | 5.00 | 4.75 |
SD | 0.50 | 0.00 | 0.71 | ||
Patency test | Mean | 3.75 | - | - | |
SD | 0.69 | - | - | ||
Bleeding control | Mean | 3.31 | - | 3.50 | |
SD | 0.59 | - | 1.00 |
The overall performance SAMS scores [Figure 3] reveal that no procedures received a rating below two (borderline), with all procedures demonstrating a consistent trend of increasing SAMS scores over time.
DISCUSSION
This overview of the anastomosis quality in the initial patient groups undergoing robotic-assisted microsurgery using a new and dedicated robot for microsurgery indicates satisfactory to good outcomes across all three procedures.
The observed decrease in the time required to perform anastomoses across all three procedures is likely attributed to the ongoing learning curve experienced by the operating surgeons. Similar decreases in anastomotic time with increased experience have been observed in clinical and preclinical studies[10-12]. Future reports are anticipated to offer additional insights into this learning curve and the eventual attainment of a plateau phase in procedural efficiency.
The SAMS scores show a slight increase over time. It is hypothesized that similar to the decrease in time needed per anastomosis, SAMS scores may further increase over time due to an increase in experience by the surgeon. This increase in SAMS score has been observed in preclinical studies[5,6,11]. In robotic-assisted general surgery, 19-128 cases for colorectal, 8-95 for bariatric, 20-48 for biliary, and 10-80 for solid organ surgery are needed to reach plateau performance[13], indicating that the plateau performance in the robotic-assisted procedures has most likely not yet been reached. This hypothesis of a future increase in SAMS score is provisional, awaiting validation with the inclusion of completed SAMS scores for the entire cohorts in future reports.
Both robotic-assisted LVA and robotic-assisted Free Flap show steady mean SAMS scores, between 3 (satisfactory) and 4 (good) across most categories. Robotic-assisted DNR shows lower scores in terms of operative flow, but good to excellent scores in terms of steadiness, tissue handling, and suture placement.
One of the primary limitations of this present study arises from the relatively small sample sizes. The reduced sample size reduces the statistical power, thereby undermining the strength and generalizability of the study’s conclusions[14].
The procedures were exclusively performed by a small group of microsurgeons, or a single microsurgeon, with extensive expertise in the field. The microsurgeons differed between procedures: LVA (SSQS), DNR (TvM), and Free Flap (RvdH, TvM, SSQS). Despite being highly trained in microsurgery, the experience with robotic-assisted microsurgery was comparatively limited. This variability, together with a steep learning curve, has already been observed in literature about robotic-assisted microsurgery[10]. This underscores the dynamic nature of surgical expertise and technology adoption, emphasizing the need for continued evaluation and reporting to evaluate the learning curve.
Even though surgical robots have become more available in hospitals worldwide in the last decades, their clinical implementation in the field of plastic and reconstructive surgery is still limited[15]. A possible barrier to this adoption is the fact that most robotic platforms are designed for endoscopic general surgery. Therefore, they lack the specific requirements for microsurgery, such as fine-tipped instruments and highly magnified visualization. The relatively high costs of purchasing and maintaining robotic systems and accompanying costs and waste of disposables are additional limitations to the adoption of robotics. Dedicated microsurgical robotic platforms such as the MUSA system have the potential to enhance the current quality of microsurgery and create new treatment possibilities by enabling superhuman precision. Large-scale studies are needed to evaluate the integration of robotic assistance in clinical care and guide future developments.
In conclusion, the assessment of anastomosis quality in the initial patient groups undergoing robotic-assisted microsurgery using the MUSA robot reveals satisfactory to good outcomes across all three procedures. The decrease in anastomosis time and increase in SAMS scores suggest an ongoing learning curve experienced by operating surgeons. Anticipated future reports will offer further insights into this learning curve and the potential attainment of a plateau phase in procedural efficiency.
DECLARATIONS
Authors’ contributions
Literature research, data curation, data analysis, and writing original draft: Debeij G
Data curation: Karavolia E
Literature research, patient recruitment, and data curation: Jonis Y
Operating surgeon and supervision: van der Hulst R
Patient recruitment, operating surgeon, protocol development, and supervision: Qiu SS
Conceived original studies, operating surgeon, and supervision: van Mulken T
All authors reviewed, edited, and approved the final version of the manuscript.
Availability of data and materials
Data are available from the corresponding author upon reasonable request.
Financial support and sponsorship
The authors received no financial support for the research, authorship, and publication of this article.
Conflicts of interest
van Mulken T is clinical advisor and shareholder in the company Microsure. van der Hulst R is shareholder in the company Microsure. The other authors declared that there are no conflicts of interest.
Ethical approval and consent to participate
Ethical approval for this study was obtained from the Medical Ethics Committee (METC) azM/UM. (LVA: NL60199.068.16/METC 162053; DNR: NL64178.068.17/METC 172045; Free Flap: NL64506.068.17/METC 172047). Informed consent: All procedures were in accordance with the Helsinki Declaration of 1975, as revised in 2008. Informed consent was obtained from all patients included in this study.
Consent for publication
Not applicable.
Copyright
© The Author(s) 2024.
REFERENCES
1. Nugent E, Joyce C, Perez-Abadia G, et al. Factors influencing microsurgical skill acquisition during a dedicated training course. Microsurgery 2012;32:649-56.
2. Lakhiani C, Fisher SM, Janhofer DE, Song DH. Ergonomics in microsurgery. J Surg Oncol 2018;118:840-4.
3. Mattos LS, Caldwell DG, Peretti G, Mora F, Guastini L, Cingolani R. Microsurgery robots: addressing the needs of high-precision surgical interventions. Swiss Med Wkly 2016;146:w14375.
4. Klodmann J, Schlenk C, Hellings-kuß A, et al. An introduction to robotically assisted surgical systems: current developments and focus areas of research. Curr Robot Rep 2021;2:321-32.
5. van Mulken TJM, Boymans CAEM, Schols RM, et al. Preclinical experience using a new robotic system created for microsurgery. Plast Reconstr Surg 2018;142:1367-76.
6. van Mulken TJM, Schols RM, Qiu SS, et al. Robotic (super) microsurgery: feasibility of a new master-slave platform in an in vivo animal model and future directions. J Surg Oncol 2018;118:826-31.
7. Chan W, Niranjan N, Ramakrishnan V. Structured assessment of microsurgery skills in the clinical setting. J Plast Reconstr Aesthet Surg 2010;63:1329-34.
8. van Mulken TJM, Wolfs JAGN, Qiu SS, et al. One-year outcomes of the first human trial on robot-assisted lymphaticovenous anastomosis for breast cancer-related lymphedema. Plast Reconstr Surg 2022;149:151-61.
9. Lalonde DH. Conceptual origins, current practice, and views of wide awake hand surgery. J Hand Surg Eur Vol 2017;42:886-95.
10. Barbon C, Grünherz L, Uyulmaz S, Giovanoli P, Lindenblatt N. Exploring the learning curve of a new robotic microsurgical system for microsurgery. JPRAS Open 2022;34:126-33.
11. Alrasheed T, Liu J, Hanasono MM, Butler CE, Selber JC. Robotic microsurgery: validating an assessment tool and plotting the learning curve. Plast Reconstr Surg 2014;134:794-803.
12. Katz RD, Taylor JA, Rosson GD, Brown PR, Singh NK. Robotics in plastic and reconstructive surgery: use of a telemanipulator slave robot to perform microvascular anastomoses. J Reconstr Microsurg 2006;22:53-7.
13. Pernar LIM, Robertson FC, Tavakkoli A, Sheu EG, Brooks DC, Smink DS. An appraisal of the learning curve in robotic general surgery. Surg Endosc 2017;31:4583-96.
14. Serdar CC, Cihan M, Yücel D, Serdar MA. Sample size, power and effect size revisited: simplified and practical approaches in pre-clinical, clinical and laboratory studies. Biochem Med (Zagreb) 2021;31:010502.
Cite This Article
How to Cite
Debeij, G.; Jonis Y.; Karavolia E.; Hulst R.; Qiu S. S.; Mulken T. Robotic-assisted microsurgery using the MUSA-robot: evaluation of the learning curve in three clinical pilot trials. Plast. Aesthet. Res. 2024, 11, 48. http://dx.doi.org/10.20517/2347-9264.2024.51
Download Citation
Export Citation File:
Type of Import
Tips on Downloading Citation
Citation Manager File Format
Type of Import
Direct Import: When the Direct Import option is selected (the default state), a dialogue box will give you the option to Save or Open the downloaded citation data. Choosing Open will either launch your citation manager or give you a choice of applications with which to use the metadata. The Save option saves the file locally for later use.
Indirect Import: When the Indirect Import option is selected, the metadata is displayed and may be copied and pasted as needed.
About This Article
Copyright
Data & Comments
Data
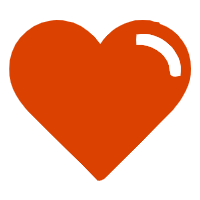
Comments
Comments must be written in English. Spam, offensive content, impersonation, and private information will not be permitted. If any comment is reported and identified as inappropriate content by OAE staff, the comment will be removed without notice. If you have any queries or need any help, please contact us at support@oaepublish.com.